Ramanujan's radical and how we define an infinite nested radicalProof of an equality involving cosine $sqrt{2...
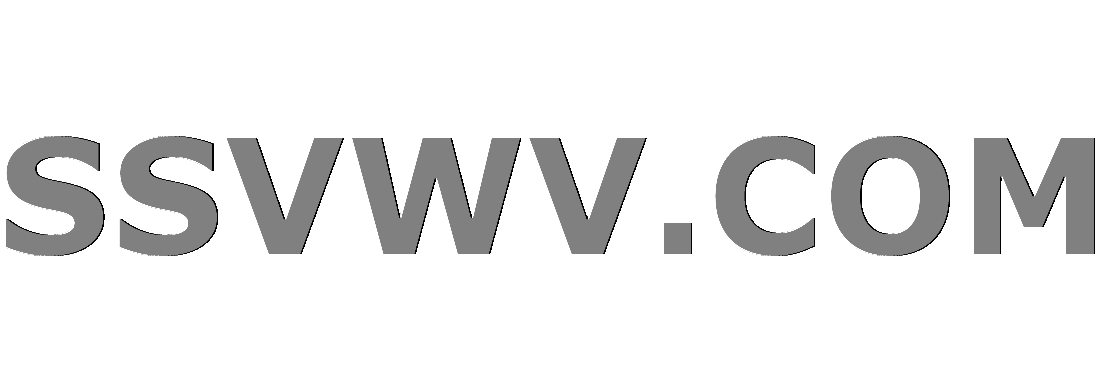
Multi tool use
Do error bars on probabilities have any meaning?
Can I use a larger HVAC Hard Start kit than is recommended?
Almost normal subgroup
Rudeness by being polite
Is there a way to draw a level tree?
Is it possible to detect 100% of SQLi with a simple regex?
Current measurement op-amp calculation
Why is quixotic not Quixotic (a proper adjective)?
Arizona laws regarding ownership of ground glassware for chemistry usage
Can I legally make a website about boycotting a certain company?
multiple null checks in Java8
Why do BLDC motor (1 kW) controllers have so many MOSFETs?
How can I add more depth to my poem?
How to know if I am a 'Real Developer'
Does changing "sa" password require a SQL restart (in mixed mode)?
Discouraging missile alpha strikes
Why do we divide Permutations to get to Combinations?
Build ASCII Podiums
Integral check. Is partial fractions the only way?
Define function that behaves almost identically to Mathematica function
Why didn't Lorentz conclude that no object can go faster than light?
Why would you use 2 alternate layout buttons instead of 1, when only one can be selected at once
Taking an academic pseudonym?
Is Screenshot Time-tracking Common?
Ramanujan's radical and how we define an infinite nested radical
Proof of an equality involving cosine $sqrt{2 + sqrt{2 + cdots + sqrt{2 + sqrt{2}}}} = 2cos (pi/2^{n+1})$Convergence of alternating nested radicalsEquality of nested radicals with different operationsIncorrect proposal to dealing with nested radicals?Ramanujan's infinitely nested radical clarificationProving Infinite Nested RadicalInfinitely nested radical expansions for real numbersDistribution of infinite nested radicals with random termsVariation to Ramanujan's infinite nested radical with primesIs The Infinite Nested Radical $sqrt{1+sqrt{x+sqrt{x^2+sqrt{x^3+…}}}}$ Analytical?How do we find the general term from an infinite nested radical
$begingroup$
I know it is true that we have
$$sqrt{1+2sqrt{1+3sqrt{1+4sqrt{1+cdots}}}}=3$$
The argument is to break the nested radical into something like
$$3 = sqrt{9}=sqrt{1+2sqrt{16}}=sqrt{1+2sqrt{1+3sqrt{25}}}=...=sqrt{1+2sqrt{1+3sqrt{1+4sqrt{1+cdots}}}}$$
However, I am not convinced. I can do something like
$$4 = sqrt{16}=sqrt{1+2sqrt{56.25}}=sqrt{1+2sqrt{1+3sqrt{frac{48841}{144}}}}=...=sqrt{1+2sqrt{1+3sqrt{1+4sqrt{1+cdots}}}}$$
Something must be wrong and and the reason behind should be misunderstanding of how we define infinte nested radical in the form of
$$ sqrt{a_{0}+a_{1}sqrt{a_{2}+a_{3}sqrt{a_{4}+a_{5}sqrt{a_{6}+cdots}}}} $$
I researched for a while but all I could find was computation tricks but not strict definition. Really need help here. Thanks.
real-analysis sequences-and-series elementary-number-theory convergence nested-radicals
$endgroup$
|
show 2 more comments
$begingroup$
I know it is true that we have
$$sqrt{1+2sqrt{1+3sqrt{1+4sqrt{1+cdots}}}}=3$$
The argument is to break the nested radical into something like
$$3 = sqrt{9}=sqrt{1+2sqrt{16}}=sqrt{1+2sqrt{1+3sqrt{25}}}=...=sqrt{1+2sqrt{1+3sqrt{1+4sqrt{1+cdots}}}}$$
However, I am not convinced. I can do something like
$$4 = sqrt{16}=sqrt{1+2sqrt{56.25}}=sqrt{1+2sqrt{1+3sqrt{frac{48841}{144}}}}=...=sqrt{1+2sqrt{1+3sqrt{1+4sqrt{1+cdots}}}}$$
Something must be wrong and and the reason behind should be misunderstanding of how we define infinte nested radical in the form of
$$ sqrt{a_{0}+a_{1}sqrt{a_{2}+a_{3}sqrt{a_{4}+a_{5}sqrt{a_{6}+cdots}}}} $$
I researched for a while but all I could find was computation tricks but not strict definition. Really need help here. Thanks.
real-analysis sequences-and-series elementary-number-theory convergence nested-radicals
$endgroup$
2
$begingroup$
Surely the rigorous definition is that it is the limit of the finite expressions that you get when you drop the $+ cdots$, provided that this limit exists.
$endgroup$
– John Coleman
yesterday
$begingroup$
So you are suggesting $sqrt{1+2sqrt{1+3sqrt{1+4sqrt{1+cdots}}}} $ actually has no universally-agreed definition in Maths?
$endgroup$
– Anson NG
yesterday
6
$begingroup$
When you write something down, you stop your writing at some stage and break into $+ cdots$. What you have written is a purely finite expression. Think of these finite expressions as terms in a sequence. What do these terms approach?
$endgroup$
– John Coleman
yesterday
2
$begingroup$
I understand your comment. Given a general term of partial sum, we can find it limit although it might not exist. We can do it because we all universally agree what it means by a general term. Sometimes the dots is clear in the sense that we would not argue its meaning. For example,comsider $ 1+2+3+4+5+...$. Someone could still argue that the 6th term can be any number and does not have to be 6 but it's kind of agreed that we will look at the pattern and take the 6th term as 6.
$endgroup$
– Anson NG
yesterday
1
$begingroup$
This is interesting since you can redefine any number this way as you pointed out. We need to think of infinite nested root with more careful definition.. Maybe it's only equal to 3 if we don't have to use fraction to expand out more nested root. That is, in the Ramanujan's sum, when you expand the nested root, they are all in term of integers. Where as, for other number, you will get fraction like term. Similar to what you have... just a thought!
$endgroup$
– user209663
yesterday
|
show 2 more comments
$begingroup$
I know it is true that we have
$$sqrt{1+2sqrt{1+3sqrt{1+4sqrt{1+cdots}}}}=3$$
The argument is to break the nested radical into something like
$$3 = sqrt{9}=sqrt{1+2sqrt{16}}=sqrt{1+2sqrt{1+3sqrt{25}}}=...=sqrt{1+2sqrt{1+3sqrt{1+4sqrt{1+cdots}}}}$$
However, I am not convinced. I can do something like
$$4 = sqrt{16}=sqrt{1+2sqrt{56.25}}=sqrt{1+2sqrt{1+3sqrt{frac{48841}{144}}}}=...=sqrt{1+2sqrt{1+3sqrt{1+4sqrt{1+cdots}}}}$$
Something must be wrong and and the reason behind should be misunderstanding of how we define infinte nested radical in the form of
$$ sqrt{a_{0}+a_{1}sqrt{a_{2}+a_{3}sqrt{a_{4}+a_{5}sqrt{a_{6}+cdots}}}} $$
I researched for a while but all I could find was computation tricks but not strict definition. Really need help here. Thanks.
real-analysis sequences-and-series elementary-number-theory convergence nested-radicals
$endgroup$
I know it is true that we have
$$sqrt{1+2sqrt{1+3sqrt{1+4sqrt{1+cdots}}}}=3$$
The argument is to break the nested radical into something like
$$3 = sqrt{9}=sqrt{1+2sqrt{16}}=sqrt{1+2sqrt{1+3sqrt{25}}}=...=sqrt{1+2sqrt{1+3sqrt{1+4sqrt{1+cdots}}}}$$
However, I am not convinced. I can do something like
$$4 = sqrt{16}=sqrt{1+2sqrt{56.25}}=sqrt{1+2sqrt{1+3sqrt{frac{48841}{144}}}}=...=sqrt{1+2sqrt{1+3sqrt{1+4sqrt{1+cdots}}}}$$
Something must be wrong and and the reason behind should be misunderstanding of how we define infinte nested radical in the form of
$$ sqrt{a_{0}+a_{1}sqrt{a_{2}+a_{3}sqrt{a_{4}+a_{5}sqrt{a_{6}+cdots}}}} $$
I researched for a while but all I could find was computation tricks but not strict definition. Really need help here. Thanks.
real-analysis sequences-and-series elementary-number-theory convergence nested-radicals
real-analysis sequences-and-series elementary-number-theory convergence nested-radicals
edited yesterday


Eevee Trainer
6,51311237
6,51311237
asked yesterday
Anson NGAnson NG
18319
18319
2
$begingroup$
Surely the rigorous definition is that it is the limit of the finite expressions that you get when you drop the $+ cdots$, provided that this limit exists.
$endgroup$
– John Coleman
yesterday
$begingroup$
So you are suggesting $sqrt{1+2sqrt{1+3sqrt{1+4sqrt{1+cdots}}}} $ actually has no universally-agreed definition in Maths?
$endgroup$
– Anson NG
yesterday
6
$begingroup$
When you write something down, you stop your writing at some stage and break into $+ cdots$. What you have written is a purely finite expression. Think of these finite expressions as terms in a sequence. What do these terms approach?
$endgroup$
– John Coleman
yesterday
2
$begingroup$
I understand your comment. Given a general term of partial sum, we can find it limit although it might not exist. We can do it because we all universally agree what it means by a general term. Sometimes the dots is clear in the sense that we would not argue its meaning. For example,comsider $ 1+2+3+4+5+...$. Someone could still argue that the 6th term can be any number and does not have to be 6 but it's kind of agreed that we will look at the pattern and take the 6th term as 6.
$endgroup$
– Anson NG
yesterday
1
$begingroup$
This is interesting since you can redefine any number this way as you pointed out. We need to think of infinite nested root with more careful definition.. Maybe it's only equal to 3 if we don't have to use fraction to expand out more nested root. That is, in the Ramanujan's sum, when you expand the nested root, they are all in term of integers. Where as, for other number, you will get fraction like term. Similar to what you have... just a thought!
$endgroup$
– user209663
yesterday
|
show 2 more comments
2
$begingroup$
Surely the rigorous definition is that it is the limit of the finite expressions that you get when you drop the $+ cdots$, provided that this limit exists.
$endgroup$
– John Coleman
yesterday
$begingroup$
So you are suggesting $sqrt{1+2sqrt{1+3sqrt{1+4sqrt{1+cdots}}}} $ actually has no universally-agreed definition in Maths?
$endgroup$
– Anson NG
yesterday
6
$begingroup$
When you write something down, you stop your writing at some stage and break into $+ cdots$. What you have written is a purely finite expression. Think of these finite expressions as terms in a sequence. What do these terms approach?
$endgroup$
– John Coleman
yesterday
2
$begingroup$
I understand your comment. Given a general term of partial sum, we can find it limit although it might not exist. We can do it because we all universally agree what it means by a general term. Sometimes the dots is clear in the sense that we would not argue its meaning. For example,comsider $ 1+2+3+4+5+...$. Someone could still argue that the 6th term can be any number and does not have to be 6 but it's kind of agreed that we will look at the pattern and take the 6th term as 6.
$endgroup$
– Anson NG
yesterday
1
$begingroup$
This is interesting since you can redefine any number this way as you pointed out. We need to think of infinite nested root with more careful definition.. Maybe it's only equal to 3 if we don't have to use fraction to expand out more nested root. That is, in the Ramanujan's sum, when you expand the nested root, they are all in term of integers. Where as, for other number, you will get fraction like term. Similar to what you have... just a thought!
$endgroup$
– user209663
yesterday
2
2
$begingroup$
Surely the rigorous definition is that it is the limit of the finite expressions that you get when you drop the $+ cdots$, provided that this limit exists.
$endgroup$
– John Coleman
yesterday
$begingroup$
Surely the rigorous definition is that it is the limit of the finite expressions that you get when you drop the $+ cdots$, provided that this limit exists.
$endgroup$
– John Coleman
yesterday
$begingroup$
So you are suggesting $sqrt{1+2sqrt{1+3sqrt{1+4sqrt{1+cdots}}}} $ actually has no universally-agreed definition in Maths?
$endgroup$
– Anson NG
yesterday
$begingroup$
So you are suggesting $sqrt{1+2sqrt{1+3sqrt{1+4sqrt{1+cdots}}}} $ actually has no universally-agreed definition in Maths?
$endgroup$
– Anson NG
yesterday
6
6
$begingroup$
When you write something down, you stop your writing at some stage and break into $+ cdots$. What you have written is a purely finite expression. Think of these finite expressions as terms in a sequence. What do these terms approach?
$endgroup$
– John Coleman
yesterday
$begingroup$
When you write something down, you stop your writing at some stage and break into $+ cdots$. What you have written is a purely finite expression. Think of these finite expressions as terms in a sequence. What do these terms approach?
$endgroup$
– John Coleman
yesterday
2
2
$begingroup$
I understand your comment. Given a general term of partial sum, we can find it limit although it might not exist. We can do it because we all universally agree what it means by a general term. Sometimes the dots is clear in the sense that we would not argue its meaning. For example,comsider $ 1+2+3+4+5+...$. Someone could still argue that the 6th term can be any number and does not have to be 6 but it's kind of agreed that we will look at the pattern and take the 6th term as 6.
$endgroup$
– Anson NG
yesterday
$begingroup$
I understand your comment. Given a general term of partial sum, we can find it limit although it might not exist. We can do it because we all universally agree what it means by a general term. Sometimes the dots is clear in the sense that we would not argue its meaning. For example,comsider $ 1+2+3+4+5+...$. Someone could still argue that the 6th term can be any number and does not have to be 6 but it's kind of agreed that we will look at the pattern and take the 6th term as 6.
$endgroup$
– Anson NG
yesterday
1
1
$begingroup$
This is interesting since you can redefine any number this way as you pointed out. We need to think of infinite nested root with more careful definition.. Maybe it's only equal to 3 if we don't have to use fraction to expand out more nested root. That is, in the Ramanujan's sum, when you expand the nested root, they are all in term of integers. Where as, for other number, you will get fraction like term. Similar to what you have... just a thought!
$endgroup$
– user209663
yesterday
$begingroup$
This is interesting since you can redefine any number this way as you pointed out. We need to think of infinite nested root with more careful definition.. Maybe it's only equal to 3 if we don't have to use fraction to expand out more nested root. That is, in the Ramanujan's sum, when you expand the nested root, they are all in term of integers. Where as, for other number, you will get fraction like term. Similar to what you have... just a thought!
$endgroup$
– user209663
yesterday
|
show 2 more comments
5 Answers
5
active
oldest
votes
$begingroup$
Introduction:
The issue is what "..." really "represents."
Typically we use it as a sort of shorthand, as if to say "look, I can't write infinitely many things down, just assume that the obvious pattern holds and goes on infinitely."
This idea holds for all sorts of things - nested radicals, infinite sums, continued fractions, infinite sequences, etc.
On Infinite Sums:
A simple example: the sum of the reciprocals of squares:
$$1 + frac{1}{4} + frac{1}{9} + frac{1}{16} + ...$$
This is a well known summation. It is the Riemann zeta function $zeta(s)$ at $s=2$, and is known to evaluate to $pi^2/6$ (proved by Euler and known as the Basel problem).
Another, easier-to-handle summation is the geometric sum
$$1 + frac 1 2 + frac 1 4 + frac 1 8 + ...$$
This is a geometric series where the ratio is $1/2$ - each summand is half the previous one. We know, too, that this evaluates to $2$.
Another geometric series you might see in proofs that $0.999... = 1$ is
$$9 left( frac{1}{10} + frac{1}{100} + frac{1}{1,000} + frac{1}{10,000} + ... right)$$
which equals $1$. In fact, any infinite geometric series, with first term $a$ and ratio $|r|<1$ can be evaluated by
$$sum_{n=0}^infty ar^n = frac{a}{1-r}$$
So a question arises - ignoring these "obvious" results (depending on your amount of mathematical knowledge), how would we know these converge to the given values? What, exactly, does it mean for a summation to converge to a number or equal a number? For finite sums this is no issue - if nothing else, we could add up each number manually, but we can't just add up every number from a set of infinitely-many numbers.
Well, one could argue by common sense that, if the sequence converges to some number, the more and more terms you add up, the closer they'll get to that number.
So we obtain one definition for the convergence of an infinite sum. Consider a sequence where the $n^{th}$ term is defined by the sum of the first $n$ terms in the sequence. To introduce some symbols, suppose we're trying to find the sum
$$sum_{k=1}^infty x_k = x_1 + x_2 + x_3 + x_4 + ...$$
for whatever these $x_i$'s are. Then define these so-called "partial sums" of this by a function $S(n)$:
$$S(n) = sum_{k=1}^n x_k = x_1 + x_2 + ... + x_n$$
Then we get a sequence of sums:
$$S(1), S(2), S(3), ...$$
or equivalently
$$x_1 ;;,;; x_1 + x_2;;,;; x_1 + x_2 + x_3;;,;; ...$$
Then we ask: what does $S(n)$ approach as $n$ grows without bound, if anything at all? (In calculus, we call this "the limit of the partial sums $S(n)$ as $n$ approaches infinity.")
For the case of our first geometric sum, we immediately see the sequence of partial sums
$$1, frac{3}{2}, frac{7}{4}, frac{15}{8},...$$
Clearly, this suggests a pattern - and if you want to, you can go ahead and prove it, I won't do so here for brevity's sake. The pattern is that the $n^{th}$ term of the sequence is
$$S(n) = frac{2^{(n+1)}-1}{2^{n}}$$
We can then easily consider the limit of these partial sums:
$$lim_{ntoinfty} S(n) = lim_{ntoinfty} frac{2^{(n+1)}-1}{2^{n}} = lim_{ntoinfty} frac{2^{(n+1)}}{2^{n}} - frac {1}{2^{n}} = lim_{ntoinfty} 2 - lim_{ntoinfty} frac{1}{2^{n}}$$
Obviously, $1/2^{n} to 0$ as $n$ grows without bound, and $2$ is not affected by $n$, so we conclude
$$lim_{ntoinfty} S(n) = lim_{ntoinfty} 2 - lim_{ntoinfty} frac{1}{2^n} = 2 - 0 = 2$$
And thus we say
$$sum_{k=0}^infty left(frac 1 2 right)^k = 1 + frac 1 2 + frac 1 4 + frac 1 8 + ... = 2$$
because the partial sums approach $2$.
On Continued Fractions:
That was a simple, "first" sort of example, but mathematicians essentially do the same thing in other contexts. I want to touch on one more such context before we deal with the radical case, just to nail that point home.
In this case, it will be with continued fractions. One of the simpler such fractions is the one for $1$:
$$1 = frac{1}{2-frac{1}{2-frac{1}{2-frac{1}{...}}}}$$
As usual, the "..." denotes that this continues forever. But what it does it mean for this infinite expression to equal $1$?
For this, we consider a more general analogue of the "partial sum" from before - a "convergent." We cut up the sequence at logical finite points, whatever those points being depending on the context. Then if the sequence of the convergents approaches a limit, we say they're equal.
What are the convergents for a continued fraction? By convention, we cut off just before the start of the next fraction. That is, in the continued fraction for $1$, we cut off at the $n^{th} ; 2$ for the $n^{th}$ convergent, and ignore what follows. So we get the sequence of convergents
$$frac{1}{2} , frac{1}{2-frac{1}{2}}, frac{1}{2-frac{1}{2-frac{1}{2}}},...$$
Working out the numbers, we find the sequence to be
$$frac{1}{2},frac{2}{3},frac{3}{4},...$$
Again, we see a pattern! The $n^{th}$ term of the sequence is clearly of the form
$$frac{n-1}{n}$$
Let $C(n)$ be a function denoting the $n^{th}$ convergent. Then $C(1)=1/2,$ $C(2) = 2/3,$ $C(n)=(n-1)/n,$ and so on. So like before we consider the infinite limit:
$$lim_{ntoinfty} C(n) = lim_{ntoinfty} frac{n-1}{n} = lim_{ntoinfty} 1 - frac 1 n = lim_{ntoinfty} 1 - lim_{ntoinfty} frac 1 n = 1 - 0 = 1$$
Thus we can conclude that the continued fraction equals $1$, because its sequence of convergents equals $1$!
On Infinite Radicals:
So now, we touch on infinite nested radicals. They're messier to deal with but doable.
One of the simpler examples of such radicals to contend with is
$$2 = sqrt{2 +sqrt{2 +sqrt{2 +sqrt{2 +sqrt{2 +...}}}}}$$
As with the previous two cases we see an infinite expression. We instinctively conclude by now: to logically define a limit for this expression - to assign it a value provided it even exists - we need to chop this up at finite points, defining a sequence of convergents $C(n)$, and then find $C(n)$ as $ntoinfty$.
Nested radicals are a lot messier than the previous, but we manage.
So first let the sequence of convergents be given by cutting off everything after the $n^{th} ; 2$ in the expression. Thus we get the sequence
$$sqrt 2 ;;,;; sqrt{2 + sqrt{2}};;,;; sqrt{2+sqrt{2+sqrt{2}}};;,;; sqrt{2+sqrt{2+sqrt{2+sqrt{2}}}}$$
Okay this isn't particularly nice already, but apparently there does exist, shockingly enough, a closed-form explicit expression for $C(n)$: (from: S. Zimmerman, C. Ho)
$$C(n) = 2cosleft(frac{pi}{2^{n+1}}right)$$
(I had to find that expression by Googling, I honestly didn't know that offhand. It can be proved by induction, as touched on in this MSE question.)
So luckily, then, we can find the limit of $C(n)$:
$$lim_{ntoinfty} C(n) = lim_{ntoinfty} 2cosleft(frac{pi}{2^{n+1}}right)$$
It is probably obvious that the argument of the cosine function approaches $0$ as $n$ grows without bound, and thus
$$lim_{ntoinfty} C(n) = lim_{ntoinfty} 2cosleft(frac{pi}{2^{n+1}}right) = 2cos(0) = 2cdot 1 = 2$$
Thus, since its convergents approach $2$, we can conclude that
$$2 = sqrt{2 +sqrt{2 +sqrt{2 +sqrt{2 +sqrt{2 +...}}}}}$$
A Lengthy Conclusion:
So, in short, how do we evaluate an infinite expression, be it radical, continued fraction, sum, or otherwise?
We begin by truncating the expression at convenient finite places, creating a series of convergents, generalizations of the "partial sums" introduced in calculus. We then try to get a closed form or some other usable expression for the convergents $C(n)$, and consider the value as $ntoinfty$. If it converges to some value, we say that the expression is in fact equal to that value. If it doesn't, then the expression doesn't converge to any value.
This doesn't mean each expression is "nice." Radical expressions in particular, in my experience, tend to be nasty as all hell, and I'm lucky I found that one closed form expression for the particularly easy radical I chose.
This doesn't mean that other methods cannot be used to find the values, so long as there's some sort of logical justification for said method. For example, there is a justification for the formula for an infinite (and finite) geometric sum. We might have to circumvent the notion of partial sums entirely, or at least it might be convenient to do so. For example, with the Basel problem, Euler's proof focused on Maclaurin series, and none of this "convergent" stuff. (That proof is here plus other proofs of it!)
Luckily, at least, this notion of convergents, even if it may not always be the best way to do it, lends itself to an easy way to check a solution to any such problem. Just find a bunch of the convergents - take as many as you need. If you somehow have multiple solutions, as you think with Ramanujan's radical, then you'll see the convergents get closer and closer to the "true" solution.
(How many convergents you need to find depends on the situation and how close your proposed solutions are. It might be immediately obvious after $10$ iterations, or might not be until $10,000,000$. This logic also relies on the assumption that there is only one solution to a given expression that is valid. Depending on the context, you might see cases where multiple solutions are valid but this "approaching by hand" method will only get you some of the solutions. This touches on the notion of "unstable" and "stable" solutions to dynamical systems - which I believe is the main context where such would pop up - but it's a bit overkill to discuss that for this post.)
So I will conclude by showing, in this way, that the solution is $3$ to Ramanujan's radical.
We begin with the radical itself:
$$sqrt{1+2sqrt{1+3sqrt{1+4sqrt{1+cdots}}}}=3$$
Let us begin by getting a series of convergents:
$$sqrt{1} ;;,;; sqrt{1 + 2sqrt{1}} ;;,;; sqrt{1 + 2sqrt{1 + 3sqrt{1}}} ;;,;;$$
Because the $sqrt{1}$ isn't necessary, we just let it be $1$.
$$1 ;;,;; sqrt{1 + 2} ;;,;; sqrt{1 + 2sqrt{1 + 3}} ;;,;;$$
Okay so ... where to go from here? Honestly, my initial temptation was to just use a MATLAB script and evaluate it, but I can't think of even a recursive closed form for this that would be nice enough. So in any event, we just have to go by "hand" (and by hand I mean WolframAlpha). Let $C(n)$ be the $n^{th}$ convergent. Then
- $C(1) = 1$
- $C(2) approx 1.732$
- $C(3) approx 2.236$
- $C(4) approx 2.560$
- $C(5) approx 2.755$
- $C(6) approx 2.867$
- $C(7) approx 2.929$
- $C(8) approx 2.963$
- $C(9) approx 2.981$
- $C(10) approx 2.990$
To skip a few values because at this point the changes get minimal, I used a macro to make a quick code for $C(50)$ so I could put it into Microsoft Excel and got the approximate result
$$C(50) approx 2.999 ; 999 ; 999 ; 999 ; 99$$
So while not the most rigorous result, we can at least on an intuitive level feel like the convergents from Ramanujan's radical converge to $3$, not $4$ or any other number. Neglecting that this is not an ironclad proof of the convergence, at least intuitively then we can feel like
$$3 = sqrt{1+2sqrt{1+3sqrt{1+4sqrt{1+cdots}}}}$$
Whew! Hopefully that lengthy post was helpful to you!
A late footnote, but Mathologer on YouTube did a video on this very topic, so his video would give a decent summary of all this stuff as well. Here's a link.
$endgroup$
3
$begingroup$
On Mathematica you can dof[x_, n_] := Sqrt[1 + n * x]; ram[x0_, n_] := Fold[f, Sqrt[x0], Reverse @ Range[2, n]];
The results suggest that the radical approaches 3 regardless of the innermost initial value.
$endgroup$
– lastresort
yesterday
add a comment |
$begingroup$
The user @Eevee Trainer provided a nice explanation on how we define infinite nested radical in terms of limit of finite nested radical which should be insensitive of the starting point. For full generality in this regard, we can consider the convergence of the following finite nested radical
$$ sqrt{1 + 2 sqrt{1 + 3 sqrt{ 1 + cdots n sqrt{1 + (n+1) a_n}}}} tag{*} $$
for a given sequence $(a_n)$ of non-negative real numbers. In this answer, I will prove the convergence of $text{(*)}$ to $3$ under a mild condition. My solution will involve some preliminary knowledge on analysis.
Setting. We consider the map $Phi$, defined on the space of all functions from $[0,infty)$ to $[0, infty)$, which is given by
$$ Phi [f](x) = sqrt{1 + xf(x+1)}. $$
Let us check how $Phi$ is related to our problem. The idea is to apply the trick of computing Ramanujan's infinite nested radical not to a single number, but rather to a function. Here, we choose $F(x) = x+1$. Since
$$ F(x) = 1+x = sqrt{1+x(x+2)} = sqrt{1 + xF(x+1)} = Phi[F](x), $$
it follows that we can iterated $Phi$ several times to obtain
$$ F(x) = Phi^{circ n}[F](x) = sqrt{1 + xsqrt{1 + (x+1)sqrt{1 + cdots (x+n-1)sqrt{(x+n+1)^2}}}}, $$
where $Phi^{circ n} = Phi circ cdots circ Phi$ is the $n$-fold function composition of $Phi$. Of course, the original radical corresponds to the case $x = 2$. This already proves that $Phi^{circ n}[F](x)$ converges to $F(x)$ as $ntoinfty$.
On the other hand, infinite nested radicals do not have any designated value to start with, and so, the above computation is far from satisfactory when it comes to defining infinite nested radical. Thus, a form of robustness of the convergence is required. In this regard, @Eevee Trainer investigated the convergence of
$$Phi^{circ n}[1](2) = sqrt{1 + 2sqrt{1 + 3sqrt{1 + cdots (n+1)sqrt{1}}}}, $$
and confirmed numerically that this still tends to $F(2) = 3$ as $n$ grows. Of course, it will be ideal if we can verify the same conclusion for other choices starting points and using rigorous argument.
Proof. One nice thing about $Phi$ is that it enjoys monotone, i.e., if $f leq g$, then $Phi [f] leq Phi [g]$. From this, it is easy to establish the following observation.
Lemma 1. For any $f geq 0$, we have $liminf_{ntoinfty} Phi^{circ n}[f](x) geq x+1$.
Proof. We inductively apply the monotonicity of $Phi$ to find that
$$Phi^{circ (n+1)} [0](x) geq x^{1-2^{-n}} qquad text{and} qquad Phi^{circ n}[x](x) geq x + 1 - 2^{-n}. $$
So, for any integer $m geq 0$,
begin{align*}
liminf_{ntoinfty} Phi^{circ n}[f](x)
&geq liminf_{ntoinfty} Phi^{circ m}[Phi^{circ (n+1)}[0]](x)
geq liminf_{ntoinfty} Phi^{circ m}[x^{1-2^{-(n-1)}}](x) \
&= Phi^{circ m}[x](x)
geq x + 1 - 2^{-m},
end{align*}
and letting $m to infty$ proves Lemma 1 as required.
Lemma 2. If $limsup_{xtoinfty} frac{log log max{e, f(x)}}{x} < log 2$, then $limsup_{ntoinfty} Phi^{circ n}[f](x) leq x+1$.
Proof. By the assumption, there exists $C > 1$ and $alpha in [0, 2)$ such that $f(x) leq C e^{alpha^x} (x + 1)$. Again, applying monotonicity of $Phi$, we check that $Phi^{circ n}[f](x) leq C^{2^{-n}} e^{(alpha/2)^n alpha^x} (x+1)$ holds. This is certainly true if $n = 0$. Moreover, assuming that this is true for $n$,
begin{align*}
Phi^{circ (n+1)}[f](x)
&leq Phileft[C^{2^{-n}} e^{(alpha/2)^n alpha^x} (x+1)right](x) \
&= left( 1 + x C^{2^{-n}} e^{(alpha/2)^n alpha^{x+1}} (x+2) right)^{1/2} \
&leq C^{2^{-n-1}} e^{(alpha/2)^{n+1} alpha^{x}} (x+1).
end{align*}
Now letting $n to infty$ proves the desired result.
Corollary. If $a_n geq 0$ satisfies $limsup_{ntoinfty} frac{loglog max{e, a_n}}{n} < log 2$, then for any $x geq 0$,
$$ lim_{ntoinfty} sqrt{1 + x sqrt{1 + (x+1) sqrt{ 1 + cdots + (x+n-2) sqrt{1 + (x+n-1) a_n }}}} = x+1. $$
Proof. Apply Lemma 1 and 2 to the function $f$ which interpolates $(a_n)$, such as using piecewise linear interpolation.
The bound in Lemma 2 and Corollary is optimal. Indeed, consider the non-example in OP's question of expanding $4$ as in Ramanujan's infinite nested radical. So we define the sequence $a_n$ so as to satisfy
$$ sqrt{1 + 2 sqrt{1 + 3 sqrt{ 1 + cdots n sqrt{1 + (n+1) a_n}}}} = 4. $$
Its first 4 terms are given as follows.
$$ a_1 = frac{15}{2}, quad
a_2 = frac{221}{12}, quad
a_3 = frac{48697}{576}, quad
a_4 = frac{2371066033}{1658880}, quad cdots. $$
Then we can show that $frac{1}{n}log log a_n to log 2$ as $n to infty$.
$endgroup$
add a comment |
$begingroup$
As others have said, the rigorous definition of an infinite expression comes from the limit of a sequence of finite terms. The terms need to be well-defined, but in practice, we just try to make sure the pattern is clear from context.
Now let's see what goes wrong with your other example. You wrote:
$$4 = sqrt{16}=sqrt{1+2sqrt{56.25}}=sqrt{1+2sqrt{1+3sqrt{frac{48841}{144}}}}=...=sqrt{1+2sqrt{1+3sqrt{1+4sqrt{1+cdots}}}}$$
The problem is that each term in the sequence (e.g. if we stop at $4$) fails to include an "extra" amount (and this amount is not going to zero). So if we look at the partial sums, we see they won't converge to $4$ unless we include the extra amounts we keep pushing to the right. It's the same logical mistake as taking
begin{align*}
2 &= 1 + 1 \
&= frac{1}{2} + frac{1}{2} + 1 \
&= frac{1}{2} + frac{1}{4} + frac{1}{4} + 1 \
&= frac{1}{2} + frac{1}{4} + frac{1}{8} + cdots + 1
end{align*}
And then saying, wait, the partial sums in the last line only converge to $1$ instead of $2$. But this is a mistake because we can't push the extra $1$ "infinitely far" right. Otherwise, the partial sum terms we write down will just look like $frac{1}{2} + frac{1}{4} + cdots$ and will never include the $1$. The same thing is happening (roughly) in your example.
$endgroup$
$begingroup$
However, the terms in the argument for the value being 3 are 16, 25, 36, ... also not converging to 0.
$endgroup$
– M.Herzkamp
23 hours ago
$begingroup$
@M.Herzkamp, I've edited to avoid that example. To look at the extra terms rigorously, you really have to subtract the entire term in the sequence from the total, which doesn't have a simple closed form in the square root examples.
$endgroup$
– usul
22 hours ago
add a comment |
$begingroup$
Your example doesn't quite capture the spirit of the Ramanujan expression: it arises from the succession of the integers, not from ad hoc computation to try to fit the expression to a value.
Let's make a sketch of an induction proof. Take the base case $n=3$:
$$sqrt{n^2}=3$$
and, for the inductive step, take the radical at the "deepest" level of the chain and apply
$$sqrt{k^2}=sqrt{1+(k-1)(k+1)}$$
$$=sqrt{1+(k-1)sqrt{(k+1)^2}}$$
this gives us exactly the sequence we need. This means we can take any $N>=3$ and construct the expression with $sqrt{N^2}$ in the deepest radical, and know that the equality with 3 has been preserved. (What does this tell us about the limit at infinity?)
For the 4 example, there's no analogous step to get from one term of the sequence to the next; you have to compute the term at the lowest level by working through the whole chain each time.
New contributor
ripkoops is a new contributor to this site. Take care in asking for clarification, commenting, and answering.
Check out our Code of Conduct.
$endgroup$
$begingroup$
Thanks for your help. However, gathering all the helps from the community so far, I would say I disagree with your points. In the case of '4' , we can definitely define a sequence of nested radicals(although the general term is super ugly) that is well-defined and converges to 4. The problem is the expression of the infinite nested radical alone is not telling us all the information. It is never properly defined by itself.
$endgroup$
– Anson NG
18 hours ago
$begingroup$
It true that your sequence converges to 4 (because that's how it's constructed), but in some sense it doesn't converge to the abstracted sequence in question here. Think about the expressionsqrt(1+2*sqrt(1+3*sqrt(1+...)))
. What's the additive contribution of the "..." part (i.e. the difference between this andsqrt(1+2*sqrt(1+3*sqrt(1)))
)? We should expect it to approach 0 as we go deeper into the chain if we want to arrive at a limit, but in your derivation you're squaring it at each term of the sequence, which offsets the reduction you'd see from it being within another square root.
$endgroup$
– ripkoops
9 hours ago
add a comment |
$begingroup$
$4=sqrt{16}=sqrt{1+3sqrt{25}}=sqrt{1+3sqrt{1+4sqrt{36}}}=sqrt{1+3sqrt{1+4sqrt{1+5sqrt{49}}}}=sqrt{1+3sqrt{1+4sqrt{1+5sqrt{1+6sqrt{64}}}}}=sqrt{1+3sqrt{1+4sqrt{1+5sqrt{1+6sqrt{1+7sqrt{81}}}}}}$
$=sqrt{1+3sqrt{1+4sqrt{1+5sqrt{1+6sqrt{1+7sqrt{1+8sqrt{1+cdots}}}}}}}$
$5=sqrt{25}=sqrt{1+4sqrt{36}}=sqrt{1+4sqrt{1+5sqrt{49}}}=sqrt{1+4sqrt{1+5sqrt{1+6sqrt{64}}}}=sqrt{1+4sqrt{1+5sqrt{1+6sqrt{1+7sqrt{81}}}}}=sqrt{1+4sqrt{1+5sqrt{1+6sqrt{1+7sqrt{1+8sqrt{100}}}}}}$
$=sqrt{1+4sqrt{1+5sqrt{1+6sqrt{1+7sqrt{1+8sqrt{1+9sqrt{1+cdots}}}}}}}$
$vdots$
$n=sqrt{1+(n-1)sqrt{1+nsqrt{1+(n+1)sqrt{1+(n+2)sqrt{1+(n+3)sqrt{1+(n+4)sqrt{1+cdots}}}}}}}$
$endgroup$
2
$begingroup$
This does not answer the question.
$endgroup$
– Wojowu
23 hours ago
2
$begingroup$
@Wojowu: however, you have to admit that it is a nice piece of information, but too much for a comment. A little explanation might be beneficial though...
$endgroup$
– M.Herzkamp
23 hours ago
add a comment |
Your Answer
StackExchange.ifUsing("editor", function () {
return StackExchange.using("mathjaxEditing", function () {
StackExchange.MarkdownEditor.creationCallbacks.add(function (editor, postfix) {
StackExchange.mathjaxEditing.prepareWmdForMathJax(editor, postfix, [["$", "$"], ["\\(","\\)"]]);
});
});
}, "mathjax-editing");
StackExchange.ready(function() {
var channelOptions = {
tags: "".split(" "),
id: "69"
};
initTagRenderer("".split(" "), "".split(" "), channelOptions);
StackExchange.using("externalEditor", function() {
// Have to fire editor after snippets, if snippets enabled
if (StackExchange.settings.snippets.snippetsEnabled) {
StackExchange.using("snippets", function() {
createEditor();
});
}
else {
createEditor();
}
});
function createEditor() {
StackExchange.prepareEditor({
heartbeatType: 'answer',
autoActivateHeartbeat: false,
convertImagesToLinks: true,
noModals: true,
showLowRepImageUploadWarning: true,
reputationToPostImages: 10,
bindNavPrevention: true,
postfix: "",
imageUploader: {
brandingHtml: "Powered by u003ca class="icon-imgur-white" href="https://imgur.com/"u003eu003c/au003e",
contentPolicyHtml: "User contributions licensed under u003ca href="https://creativecommons.org/licenses/by-sa/3.0/"u003ecc by-sa 3.0 with attribution requiredu003c/au003e u003ca href="https://stackoverflow.com/legal/content-policy"u003e(content policy)u003c/au003e",
allowUrls: true
},
noCode: true, onDemand: true,
discardSelector: ".discard-answer"
,immediatelyShowMarkdownHelp:true
});
}
});
Sign up or log in
StackExchange.ready(function () {
StackExchange.helpers.onClickDraftSave('#login-link');
});
Sign up using Google
Sign up using Facebook
Sign up using Email and Password
Post as a guest
Required, but never shown
StackExchange.ready(
function () {
StackExchange.openid.initPostLogin('.new-post-login', 'https%3a%2f%2fmath.stackexchange.com%2fquestions%2f3119631%2framanujans-radical-and-how-we-define-an-infinite-nested-radical%23new-answer', 'question_page');
}
);
Post as a guest
Required, but never shown
5 Answers
5
active
oldest
votes
5 Answers
5
active
oldest
votes
active
oldest
votes
active
oldest
votes
$begingroup$
Introduction:
The issue is what "..." really "represents."
Typically we use it as a sort of shorthand, as if to say "look, I can't write infinitely many things down, just assume that the obvious pattern holds and goes on infinitely."
This idea holds for all sorts of things - nested radicals, infinite sums, continued fractions, infinite sequences, etc.
On Infinite Sums:
A simple example: the sum of the reciprocals of squares:
$$1 + frac{1}{4} + frac{1}{9} + frac{1}{16} + ...$$
This is a well known summation. It is the Riemann zeta function $zeta(s)$ at $s=2$, and is known to evaluate to $pi^2/6$ (proved by Euler and known as the Basel problem).
Another, easier-to-handle summation is the geometric sum
$$1 + frac 1 2 + frac 1 4 + frac 1 8 + ...$$
This is a geometric series where the ratio is $1/2$ - each summand is half the previous one. We know, too, that this evaluates to $2$.
Another geometric series you might see in proofs that $0.999... = 1$ is
$$9 left( frac{1}{10} + frac{1}{100} + frac{1}{1,000} + frac{1}{10,000} + ... right)$$
which equals $1$. In fact, any infinite geometric series, with first term $a$ and ratio $|r|<1$ can be evaluated by
$$sum_{n=0}^infty ar^n = frac{a}{1-r}$$
So a question arises - ignoring these "obvious" results (depending on your amount of mathematical knowledge), how would we know these converge to the given values? What, exactly, does it mean for a summation to converge to a number or equal a number? For finite sums this is no issue - if nothing else, we could add up each number manually, but we can't just add up every number from a set of infinitely-many numbers.
Well, one could argue by common sense that, if the sequence converges to some number, the more and more terms you add up, the closer they'll get to that number.
So we obtain one definition for the convergence of an infinite sum. Consider a sequence where the $n^{th}$ term is defined by the sum of the first $n$ terms in the sequence. To introduce some symbols, suppose we're trying to find the sum
$$sum_{k=1}^infty x_k = x_1 + x_2 + x_3 + x_4 + ...$$
for whatever these $x_i$'s are. Then define these so-called "partial sums" of this by a function $S(n)$:
$$S(n) = sum_{k=1}^n x_k = x_1 + x_2 + ... + x_n$$
Then we get a sequence of sums:
$$S(1), S(2), S(3), ...$$
or equivalently
$$x_1 ;;,;; x_1 + x_2;;,;; x_1 + x_2 + x_3;;,;; ...$$
Then we ask: what does $S(n)$ approach as $n$ grows without bound, if anything at all? (In calculus, we call this "the limit of the partial sums $S(n)$ as $n$ approaches infinity.")
For the case of our first geometric sum, we immediately see the sequence of partial sums
$$1, frac{3}{2}, frac{7}{4}, frac{15}{8},...$$
Clearly, this suggests a pattern - and if you want to, you can go ahead and prove it, I won't do so here for brevity's sake. The pattern is that the $n^{th}$ term of the sequence is
$$S(n) = frac{2^{(n+1)}-1}{2^{n}}$$
We can then easily consider the limit of these partial sums:
$$lim_{ntoinfty} S(n) = lim_{ntoinfty} frac{2^{(n+1)}-1}{2^{n}} = lim_{ntoinfty} frac{2^{(n+1)}}{2^{n}} - frac {1}{2^{n}} = lim_{ntoinfty} 2 - lim_{ntoinfty} frac{1}{2^{n}}$$
Obviously, $1/2^{n} to 0$ as $n$ grows without bound, and $2$ is not affected by $n$, so we conclude
$$lim_{ntoinfty} S(n) = lim_{ntoinfty} 2 - lim_{ntoinfty} frac{1}{2^n} = 2 - 0 = 2$$
And thus we say
$$sum_{k=0}^infty left(frac 1 2 right)^k = 1 + frac 1 2 + frac 1 4 + frac 1 8 + ... = 2$$
because the partial sums approach $2$.
On Continued Fractions:
That was a simple, "first" sort of example, but mathematicians essentially do the same thing in other contexts. I want to touch on one more such context before we deal with the radical case, just to nail that point home.
In this case, it will be with continued fractions. One of the simpler such fractions is the one for $1$:
$$1 = frac{1}{2-frac{1}{2-frac{1}{2-frac{1}{...}}}}$$
As usual, the "..." denotes that this continues forever. But what it does it mean for this infinite expression to equal $1$?
For this, we consider a more general analogue of the "partial sum" from before - a "convergent." We cut up the sequence at logical finite points, whatever those points being depending on the context. Then if the sequence of the convergents approaches a limit, we say they're equal.
What are the convergents for a continued fraction? By convention, we cut off just before the start of the next fraction. That is, in the continued fraction for $1$, we cut off at the $n^{th} ; 2$ for the $n^{th}$ convergent, and ignore what follows. So we get the sequence of convergents
$$frac{1}{2} , frac{1}{2-frac{1}{2}}, frac{1}{2-frac{1}{2-frac{1}{2}}},...$$
Working out the numbers, we find the sequence to be
$$frac{1}{2},frac{2}{3},frac{3}{4},...$$
Again, we see a pattern! The $n^{th}$ term of the sequence is clearly of the form
$$frac{n-1}{n}$$
Let $C(n)$ be a function denoting the $n^{th}$ convergent. Then $C(1)=1/2,$ $C(2) = 2/3,$ $C(n)=(n-1)/n,$ and so on. So like before we consider the infinite limit:
$$lim_{ntoinfty} C(n) = lim_{ntoinfty} frac{n-1}{n} = lim_{ntoinfty} 1 - frac 1 n = lim_{ntoinfty} 1 - lim_{ntoinfty} frac 1 n = 1 - 0 = 1$$
Thus we can conclude that the continued fraction equals $1$, because its sequence of convergents equals $1$!
On Infinite Radicals:
So now, we touch on infinite nested radicals. They're messier to deal with but doable.
One of the simpler examples of such radicals to contend with is
$$2 = sqrt{2 +sqrt{2 +sqrt{2 +sqrt{2 +sqrt{2 +...}}}}}$$
As with the previous two cases we see an infinite expression. We instinctively conclude by now: to logically define a limit for this expression - to assign it a value provided it even exists - we need to chop this up at finite points, defining a sequence of convergents $C(n)$, and then find $C(n)$ as $ntoinfty$.
Nested radicals are a lot messier than the previous, but we manage.
So first let the sequence of convergents be given by cutting off everything after the $n^{th} ; 2$ in the expression. Thus we get the sequence
$$sqrt 2 ;;,;; sqrt{2 + sqrt{2}};;,;; sqrt{2+sqrt{2+sqrt{2}}};;,;; sqrt{2+sqrt{2+sqrt{2+sqrt{2}}}}$$
Okay this isn't particularly nice already, but apparently there does exist, shockingly enough, a closed-form explicit expression for $C(n)$: (from: S. Zimmerman, C. Ho)
$$C(n) = 2cosleft(frac{pi}{2^{n+1}}right)$$
(I had to find that expression by Googling, I honestly didn't know that offhand. It can be proved by induction, as touched on in this MSE question.)
So luckily, then, we can find the limit of $C(n)$:
$$lim_{ntoinfty} C(n) = lim_{ntoinfty} 2cosleft(frac{pi}{2^{n+1}}right)$$
It is probably obvious that the argument of the cosine function approaches $0$ as $n$ grows without bound, and thus
$$lim_{ntoinfty} C(n) = lim_{ntoinfty} 2cosleft(frac{pi}{2^{n+1}}right) = 2cos(0) = 2cdot 1 = 2$$
Thus, since its convergents approach $2$, we can conclude that
$$2 = sqrt{2 +sqrt{2 +sqrt{2 +sqrt{2 +sqrt{2 +...}}}}}$$
A Lengthy Conclusion:
So, in short, how do we evaluate an infinite expression, be it radical, continued fraction, sum, or otherwise?
We begin by truncating the expression at convenient finite places, creating a series of convergents, generalizations of the "partial sums" introduced in calculus. We then try to get a closed form or some other usable expression for the convergents $C(n)$, and consider the value as $ntoinfty$. If it converges to some value, we say that the expression is in fact equal to that value. If it doesn't, then the expression doesn't converge to any value.
This doesn't mean each expression is "nice." Radical expressions in particular, in my experience, tend to be nasty as all hell, and I'm lucky I found that one closed form expression for the particularly easy radical I chose.
This doesn't mean that other methods cannot be used to find the values, so long as there's some sort of logical justification for said method. For example, there is a justification for the formula for an infinite (and finite) geometric sum. We might have to circumvent the notion of partial sums entirely, or at least it might be convenient to do so. For example, with the Basel problem, Euler's proof focused on Maclaurin series, and none of this "convergent" stuff. (That proof is here plus other proofs of it!)
Luckily, at least, this notion of convergents, even if it may not always be the best way to do it, lends itself to an easy way to check a solution to any such problem. Just find a bunch of the convergents - take as many as you need. If you somehow have multiple solutions, as you think with Ramanujan's radical, then you'll see the convergents get closer and closer to the "true" solution.
(How many convergents you need to find depends on the situation and how close your proposed solutions are. It might be immediately obvious after $10$ iterations, or might not be until $10,000,000$. This logic also relies on the assumption that there is only one solution to a given expression that is valid. Depending on the context, you might see cases where multiple solutions are valid but this "approaching by hand" method will only get you some of the solutions. This touches on the notion of "unstable" and "stable" solutions to dynamical systems - which I believe is the main context where such would pop up - but it's a bit overkill to discuss that for this post.)
So I will conclude by showing, in this way, that the solution is $3$ to Ramanujan's radical.
We begin with the radical itself:
$$sqrt{1+2sqrt{1+3sqrt{1+4sqrt{1+cdots}}}}=3$$
Let us begin by getting a series of convergents:
$$sqrt{1} ;;,;; sqrt{1 + 2sqrt{1}} ;;,;; sqrt{1 + 2sqrt{1 + 3sqrt{1}}} ;;,;;$$
Because the $sqrt{1}$ isn't necessary, we just let it be $1$.
$$1 ;;,;; sqrt{1 + 2} ;;,;; sqrt{1 + 2sqrt{1 + 3}} ;;,;;$$
Okay so ... where to go from here? Honestly, my initial temptation was to just use a MATLAB script and evaluate it, but I can't think of even a recursive closed form for this that would be nice enough. So in any event, we just have to go by "hand" (and by hand I mean WolframAlpha). Let $C(n)$ be the $n^{th}$ convergent. Then
- $C(1) = 1$
- $C(2) approx 1.732$
- $C(3) approx 2.236$
- $C(4) approx 2.560$
- $C(5) approx 2.755$
- $C(6) approx 2.867$
- $C(7) approx 2.929$
- $C(8) approx 2.963$
- $C(9) approx 2.981$
- $C(10) approx 2.990$
To skip a few values because at this point the changes get minimal, I used a macro to make a quick code for $C(50)$ so I could put it into Microsoft Excel and got the approximate result
$$C(50) approx 2.999 ; 999 ; 999 ; 999 ; 99$$
So while not the most rigorous result, we can at least on an intuitive level feel like the convergents from Ramanujan's radical converge to $3$, not $4$ or any other number. Neglecting that this is not an ironclad proof of the convergence, at least intuitively then we can feel like
$$3 = sqrt{1+2sqrt{1+3sqrt{1+4sqrt{1+cdots}}}}$$
Whew! Hopefully that lengthy post was helpful to you!
A late footnote, but Mathologer on YouTube did a video on this very topic, so his video would give a decent summary of all this stuff as well. Here's a link.
$endgroup$
3
$begingroup$
On Mathematica you can dof[x_, n_] := Sqrt[1 + n * x]; ram[x0_, n_] := Fold[f, Sqrt[x0], Reverse @ Range[2, n]];
The results suggest that the radical approaches 3 regardless of the innermost initial value.
$endgroup$
– lastresort
yesterday
add a comment |
$begingroup$
Introduction:
The issue is what "..." really "represents."
Typically we use it as a sort of shorthand, as if to say "look, I can't write infinitely many things down, just assume that the obvious pattern holds and goes on infinitely."
This idea holds for all sorts of things - nested radicals, infinite sums, continued fractions, infinite sequences, etc.
On Infinite Sums:
A simple example: the sum of the reciprocals of squares:
$$1 + frac{1}{4} + frac{1}{9} + frac{1}{16} + ...$$
This is a well known summation. It is the Riemann zeta function $zeta(s)$ at $s=2$, and is known to evaluate to $pi^2/6$ (proved by Euler and known as the Basel problem).
Another, easier-to-handle summation is the geometric sum
$$1 + frac 1 2 + frac 1 4 + frac 1 8 + ...$$
This is a geometric series where the ratio is $1/2$ - each summand is half the previous one. We know, too, that this evaluates to $2$.
Another geometric series you might see in proofs that $0.999... = 1$ is
$$9 left( frac{1}{10} + frac{1}{100} + frac{1}{1,000} + frac{1}{10,000} + ... right)$$
which equals $1$. In fact, any infinite geometric series, with first term $a$ and ratio $|r|<1$ can be evaluated by
$$sum_{n=0}^infty ar^n = frac{a}{1-r}$$
So a question arises - ignoring these "obvious" results (depending on your amount of mathematical knowledge), how would we know these converge to the given values? What, exactly, does it mean for a summation to converge to a number or equal a number? For finite sums this is no issue - if nothing else, we could add up each number manually, but we can't just add up every number from a set of infinitely-many numbers.
Well, one could argue by common sense that, if the sequence converges to some number, the more and more terms you add up, the closer they'll get to that number.
So we obtain one definition for the convergence of an infinite sum. Consider a sequence where the $n^{th}$ term is defined by the sum of the first $n$ terms in the sequence. To introduce some symbols, suppose we're trying to find the sum
$$sum_{k=1}^infty x_k = x_1 + x_2 + x_3 + x_4 + ...$$
for whatever these $x_i$'s are. Then define these so-called "partial sums" of this by a function $S(n)$:
$$S(n) = sum_{k=1}^n x_k = x_1 + x_2 + ... + x_n$$
Then we get a sequence of sums:
$$S(1), S(2), S(3), ...$$
or equivalently
$$x_1 ;;,;; x_1 + x_2;;,;; x_1 + x_2 + x_3;;,;; ...$$
Then we ask: what does $S(n)$ approach as $n$ grows without bound, if anything at all? (In calculus, we call this "the limit of the partial sums $S(n)$ as $n$ approaches infinity.")
For the case of our first geometric sum, we immediately see the sequence of partial sums
$$1, frac{3}{2}, frac{7}{4}, frac{15}{8},...$$
Clearly, this suggests a pattern - and if you want to, you can go ahead and prove it, I won't do so here for brevity's sake. The pattern is that the $n^{th}$ term of the sequence is
$$S(n) = frac{2^{(n+1)}-1}{2^{n}}$$
We can then easily consider the limit of these partial sums:
$$lim_{ntoinfty} S(n) = lim_{ntoinfty} frac{2^{(n+1)}-1}{2^{n}} = lim_{ntoinfty} frac{2^{(n+1)}}{2^{n}} - frac {1}{2^{n}} = lim_{ntoinfty} 2 - lim_{ntoinfty} frac{1}{2^{n}}$$
Obviously, $1/2^{n} to 0$ as $n$ grows without bound, and $2$ is not affected by $n$, so we conclude
$$lim_{ntoinfty} S(n) = lim_{ntoinfty} 2 - lim_{ntoinfty} frac{1}{2^n} = 2 - 0 = 2$$
And thus we say
$$sum_{k=0}^infty left(frac 1 2 right)^k = 1 + frac 1 2 + frac 1 4 + frac 1 8 + ... = 2$$
because the partial sums approach $2$.
On Continued Fractions:
That was a simple, "first" sort of example, but mathematicians essentially do the same thing in other contexts. I want to touch on one more such context before we deal with the radical case, just to nail that point home.
In this case, it will be with continued fractions. One of the simpler such fractions is the one for $1$:
$$1 = frac{1}{2-frac{1}{2-frac{1}{2-frac{1}{...}}}}$$
As usual, the "..." denotes that this continues forever. But what it does it mean for this infinite expression to equal $1$?
For this, we consider a more general analogue of the "partial sum" from before - a "convergent." We cut up the sequence at logical finite points, whatever those points being depending on the context. Then if the sequence of the convergents approaches a limit, we say they're equal.
What are the convergents for a continued fraction? By convention, we cut off just before the start of the next fraction. That is, in the continued fraction for $1$, we cut off at the $n^{th} ; 2$ for the $n^{th}$ convergent, and ignore what follows. So we get the sequence of convergents
$$frac{1}{2} , frac{1}{2-frac{1}{2}}, frac{1}{2-frac{1}{2-frac{1}{2}}},...$$
Working out the numbers, we find the sequence to be
$$frac{1}{2},frac{2}{3},frac{3}{4},...$$
Again, we see a pattern! The $n^{th}$ term of the sequence is clearly of the form
$$frac{n-1}{n}$$
Let $C(n)$ be a function denoting the $n^{th}$ convergent. Then $C(1)=1/2,$ $C(2) = 2/3,$ $C(n)=(n-1)/n,$ and so on. So like before we consider the infinite limit:
$$lim_{ntoinfty} C(n) = lim_{ntoinfty} frac{n-1}{n} = lim_{ntoinfty} 1 - frac 1 n = lim_{ntoinfty} 1 - lim_{ntoinfty} frac 1 n = 1 - 0 = 1$$
Thus we can conclude that the continued fraction equals $1$, because its sequence of convergents equals $1$!
On Infinite Radicals:
So now, we touch on infinite nested radicals. They're messier to deal with but doable.
One of the simpler examples of such radicals to contend with is
$$2 = sqrt{2 +sqrt{2 +sqrt{2 +sqrt{2 +sqrt{2 +...}}}}}$$
As with the previous two cases we see an infinite expression. We instinctively conclude by now: to logically define a limit for this expression - to assign it a value provided it even exists - we need to chop this up at finite points, defining a sequence of convergents $C(n)$, and then find $C(n)$ as $ntoinfty$.
Nested radicals are a lot messier than the previous, but we manage.
So first let the sequence of convergents be given by cutting off everything after the $n^{th} ; 2$ in the expression. Thus we get the sequence
$$sqrt 2 ;;,;; sqrt{2 + sqrt{2}};;,;; sqrt{2+sqrt{2+sqrt{2}}};;,;; sqrt{2+sqrt{2+sqrt{2+sqrt{2}}}}$$
Okay this isn't particularly nice already, but apparently there does exist, shockingly enough, a closed-form explicit expression for $C(n)$: (from: S. Zimmerman, C. Ho)
$$C(n) = 2cosleft(frac{pi}{2^{n+1}}right)$$
(I had to find that expression by Googling, I honestly didn't know that offhand. It can be proved by induction, as touched on in this MSE question.)
So luckily, then, we can find the limit of $C(n)$:
$$lim_{ntoinfty} C(n) = lim_{ntoinfty} 2cosleft(frac{pi}{2^{n+1}}right)$$
It is probably obvious that the argument of the cosine function approaches $0$ as $n$ grows without bound, and thus
$$lim_{ntoinfty} C(n) = lim_{ntoinfty} 2cosleft(frac{pi}{2^{n+1}}right) = 2cos(0) = 2cdot 1 = 2$$
Thus, since its convergents approach $2$, we can conclude that
$$2 = sqrt{2 +sqrt{2 +sqrt{2 +sqrt{2 +sqrt{2 +...}}}}}$$
A Lengthy Conclusion:
So, in short, how do we evaluate an infinite expression, be it radical, continued fraction, sum, or otherwise?
We begin by truncating the expression at convenient finite places, creating a series of convergents, generalizations of the "partial sums" introduced in calculus. We then try to get a closed form or some other usable expression for the convergents $C(n)$, and consider the value as $ntoinfty$. If it converges to some value, we say that the expression is in fact equal to that value. If it doesn't, then the expression doesn't converge to any value.
This doesn't mean each expression is "nice." Radical expressions in particular, in my experience, tend to be nasty as all hell, and I'm lucky I found that one closed form expression for the particularly easy radical I chose.
This doesn't mean that other methods cannot be used to find the values, so long as there's some sort of logical justification for said method. For example, there is a justification for the formula for an infinite (and finite) geometric sum. We might have to circumvent the notion of partial sums entirely, or at least it might be convenient to do so. For example, with the Basel problem, Euler's proof focused on Maclaurin series, and none of this "convergent" stuff. (That proof is here plus other proofs of it!)
Luckily, at least, this notion of convergents, even if it may not always be the best way to do it, lends itself to an easy way to check a solution to any such problem. Just find a bunch of the convergents - take as many as you need. If you somehow have multiple solutions, as you think with Ramanujan's radical, then you'll see the convergents get closer and closer to the "true" solution.
(How many convergents you need to find depends on the situation and how close your proposed solutions are. It might be immediately obvious after $10$ iterations, or might not be until $10,000,000$. This logic also relies on the assumption that there is only one solution to a given expression that is valid. Depending on the context, you might see cases where multiple solutions are valid but this "approaching by hand" method will only get you some of the solutions. This touches on the notion of "unstable" and "stable" solutions to dynamical systems - which I believe is the main context where such would pop up - but it's a bit overkill to discuss that for this post.)
So I will conclude by showing, in this way, that the solution is $3$ to Ramanujan's radical.
We begin with the radical itself:
$$sqrt{1+2sqrt{1+3sqrt{1+4sqrt{1+cdots}}}}=3$$
Let us begin by getting a series of convergents:
$$sqrt{1} ;;,;; sqrt{1 + 2sqrt{1}} ;;,;; sqrt{1 + 2sqrt{1 + 3sqrt{1}}} ;;,;;$$
Because the $sqrt{1}$ isn't necessary, we just let it be $1$.
$$1 ;;,;; sqrt{1 + 2} ;;,;; sqrt{1 + 2sqrt{1 + 3}} ;;,;;$$
Okay so ... where to go from here? Honestly, my initial temptation was to just use a MATLAB script and evaluate it, but I can't think of even a recursive closed form for this that would be nice enough. So in any event, we just have to go by "hand" (and by hand I mean WolframAlpha). Let $C(n)$ be the $n^{th}$ convergent. Then
- $C(1) = 1$
- $C(2) approx 1.732$
- $C(3) approx 2.236$
- $C(4) approx 2.560$
- $C(5) approx 2.755$
- $C(6) approx 2.867$
- $C(7) approx 2.929$
- $C(8) approx 2.963$
- $C(9) approx 2.981$
- $C(10) approx 2.990$
To skip a few values because at this point the changes get minimal, I used a macro to make a quick code for $C(50)$ so I could put it into Microsoft Excel and got the approximate result
$$C(50) approx 2.999 ; 999 ; 999 ; 999 ; 99$$
So while not the most rigorous result, we can at least on an intuitive level feel like the convergents from Ramanujan's radical converge to $3$, not $4$ or any other number. Neglecting that this is not an ironclad proof of the convergence, at least intuitively then we can feel like
$$3 = sqrt{1+2sqrt{1+3sqrt{1+4sqrt{1+cdots}}}}$$
Whew! Hopefully that lengthy post was helpful to you!
A late footnote, but Mathologer on YouTube did a video on this very topic, so his video would give a decent summary of all this stuff as well. Here's a link.
$endgroup$
3
$begingroup$
On Mathematica you can dof[x_, n_] := Sqrt[1 + n * x]; ram[x0_, n_] := Fold[f, Sqrt[x0], Reverse @ Range[2, n]];
The results suggest that the radical approaches 3 regardless of the innermost initial value.
$endgroup$
– lastresort
yesterday
add a comment |
$begingroup$
Introduction:
The issue is what "..." really "represents."
Typically we use it as a sort of shorthand, as if to say "look, I can't write infinitely many things down, just assume that the obvious pattern holds and goes on infinitely."
This idea holds for all sorts of things - nested radicals, infinite sums, continued fractions, infinite sequences, etc.
On Infinite Sums:
A simple example: the sum of the reciprocals of squares:
$$1 + frac{1}{4} + frac{1}{9} + frac{1}{16} + ...$$
This is a well known summation. It is the Riemann zeta function $zeta(s)$ at $s=2$, and is known to evaluate to $pi^2/6$ (proved by Euler and known as the Basel problem).
Another, easier-to-handle summation is the geometric sum
$$1 + frac 1 2 + frac 1 4 + frac 1 8 + ...$$
This is a geometric series where the ratio is $1/2$ - each summand is half the previous one. We know, too, that this evaluates to $2$.
Another geometric series you might see in proofs that $0.999... = 1$ is
$$9 left( frac{1}{10} + frac{1}{100} + frac{1}{1,000} + frac{1}{10,000} + ... right)$$
which equals $1$. In fact, any infinite geometric series, with first term $a$ and ratio $|r|<1$ can be evaluated by
$$sum_{n=0}^infty ar^n = frac{a}{1-r}$$
So a question arises - ignoring these "obvious" results (depending on your amount of mathematical knowledge), how would we know these converge to the given values? What, exactly, does it mean for a summation to converge to a number or equal a number? For finite sums this is no issue - if nothing else, we could add up each number manually, but we can't just add up every number from a set of infinitely-many numbers.
Well, one could argue by common sense that, if the sequence converges to some number, the more and more terms you add up, the closer they'll get to that number.
So we obtain one definition for the convergence of an infinite sum. Consider a sequence where the $n^{th}$ term is defined by the sum of the first $n$ terms in the sequence. To introduce some symbols, suppose we're trying to find the sum
$$sum_{k=1}^infty x_k = x_1 + x_2 + x_3 + x_4 + ...$$
for whatever these $x_i$'s are. Then define these so-called "partial sums" of this by a function $S(n)$:
$$S(n) = sum_{k=1}^n x_k = x_1 + x_2 + ... + x_n$$
Then we get a sequence of sums:
$$S(1), S(2), S(3), ...$$
or equivalently
$$x_1 ;;,;; x_1 + x_2;;,;; x_1 + x_2 + x_3;;,;; ...$$
Then we ask: what does $S(n)$ approach as $n$ grows without bound, if anything at all? (In calculus, we call this "the limit of the partial sums $S(n)$ as $n$ approaches infinity.")
For the case of our first geometric sum, we immediately see the sequence of partial sums
$$1, frac{3}{2}, frac{7}{4}, frac{15}{8},...$$
Clearly, this suggests a pattern - and if you want to, you can go ahead and prove it, I won't do so here for brevity's sake. The pattern is that the $n^{th}$ term of the sequence is
$$S(n) = frac{2^{(n+1)}-1}{2^{n}}$$
We can then easily consider the limit of these partial sums:
$$lim_{ntoinfty} S(n) = lim_{ntoinfty} frac{2^{(n+1)}-1}{2^{n}} = lim_{ntoinfty} frac{2^{(n+1)}}{2^{n}} - frac {1}{2^{n}} = lim_{ntoinfty} 2 - lim_{ntoinfty} frac{1}{2^{n}}$$
Obviously, $1/2^{n} to 0$ as $n$ grows without bound, and $2$ is not affected by $n$, so we conclude
$$lim_{ntoinfty} S(n) = lim_{ntoinfty} 2 - lim_{ntoinfty} frac{1}{2^n} = 2 - 0 = 2$$
And thus we say
$$sum_{k=0}^infty left(frac 1 2 right)^k = 1 + frac 1 2 + frac 1 4 + frac 1 8 + ... = 2$$
because the partial sums approach $2$.
On Continued Fractions:
That was a simple, "first" sort of example, but mathematicians essentially do the same thing in other contexts. I want to touch on one more such context before we deal with the radical case, just to nail that point home.
In this case, it will be with continued fractions. One of the simpler such fractions is the one for $1$:
$$1 = frac{1}{2-frac{1}{2-frac{1}{2-frac{1}{...}}}}$$
As usual, the "..." denotes that this continues forever. But what it does it mean for this infinite expression to equal $1$?
For this, we consider a more general analogue of the "partial sum" from before - a "convergent." We cut up the sequence at logical finite points, whatever those points being depending on the context. Then if the sequence of the convergents approaches a limit, we say they're equal.
What are the convergents for a continued fraction? By convention, we cut off just before the start of the next fraction. That is, in the continued fraction for $1$, we cut off at the $n^{th} ; 2$ for the $n^{th}$ convergent, and ignore what follows. So we get the sequence of convergents
$$frac{1}{2} , frac{1}{2-frac{1}{2}}, frac{1}{2-frac{1}{2-frac{1}{2}}},...$$
Working out the numbers, we find the sequence to be
$$frac{1}{2},frac{2}{3},frac{3}{4},...$$
Again, we see a pattern! The $n^{th}$ term of the sequence is clearly of the form
$$frac{n-1}{n}$$
Let $C(n)$ be a function denoting the $n^{th}$ convergent. Then $C(1)=1/2,$ $C(2) = 2/3,$ $C(n)=(n-1)/n,$ and so on. So like before we consider the infinite limit:
$$lim_{ntoinfty} C(n) = lim_{ntoinfty} frac{n-1}{n} = lim_{ntoinfty} 1 - frac 1 n = lim_{ntoinfty} 1 - lim_{ntoinfty} frac 1 n = 1 - 0 = 1$$
Thus we can conclude that the continued fraction equals $1$, because its sequence of convergents equals $1$!
On Infinite Radicals:
So now, we touch on infinite nested radicals. They're messier to deal with but doable.
One of the simpler examples of such radicals to contend with is
$$2 = sqrt{2 +sqrt{2 +sqrt{2 +sqrt{2 +sqrt{2 +...}}}}}$$
As with the previous two cases we see an infinite expression. We instinctively conclude by now: to logically define a limit for this expression - to assign it a value provided it even exists - we need to chop this up at finite points, defining a sequence of convergents $C(n)$, and then find $C(n)$ as $ntoinfty$.
Nested radicals are a lot messier than the previous, but we manage.
So first let the sequence of convergents be given by cutting off everything after the $n^{th} ; 2$ in the expression. Thus we get the sequence
$$sqrt 2 ;;,;; sqrt{2 + sqrt{2}};;,;; sqrt{2+sqrt{2+sqrt{2}}};;,;; sqrt{2+sqrt{2+sqrt{2+sqrt{2}}}}$$
Okay this isn't particularly nice already, but apparently there does exist, shockingly enough, a closed-form explicit expression for $C(n)$: (from: S. Zimmerman, C. Ho)
$$C(n) = 2cosleft(frac{pi}{2^{n+1}}right)$$
(I had to find that expression by Googling, I honestly didn't know that offhand. It can be proved by induction, as touched on in this MSE question.)
So luckily, then, we can find the limit of $C(n)$:
$$lim_{ntoinfty} C(n) = lim_{ntoinfty} 2cosleft(frac{pi}{2^{n+1}}right)$$
It is probably obvious that the argument of the cosine function approaches $0$ as $n$ grows without bound, and thus
$$lim_{ntoinfty} C(n) = lim_{ntoinfty} 2cosleft(frac{pi}{2^{n+1}}right) = 2cos(0) = 2cdot 1 = 2$$
Thus, since its convergents approach $2$, we can conclude that
$$2 = sqrt{2 +sqrt{2 +sqrt{2 +sqrt{2 +sqrt{2 +...}}}}}$$
A Lengthy Conclusion:
So, in short, how do we evaluate an infinite expression, be it radical, continued fraction, sum, or otherwise?
We begin by truncating the expression at convenient finite places, creating a series of convergents, generalizations of the "partial sums" introduced in calculus. We then try to get a closed form or some other usable expression for the convergents $C(n)$, and consider the value as $ntoinfty$. If it converges to some value, we say that the expression is in fact equal to that value. If it doesn't, then the expression doesn't converge to any value.
This doesn't mean each expression is "nice." Radical expressions in particular, in my experience, tend to be nasty as all hell, and I'm lucky I found that one closed form expression for the particularly easy radical I chose.
This doesn't mean that other methods cannot be used to find the values, so long as there's some sort of logical justification for said method. For example, there is a justification for the formula for an infinite (and finite) geometric sum. We might have to circumvent the notion of partial sums entirely, or at least it might be convenient to do so. For example, with the Basel problem, Euler's proof focused on Maclaurin series, and none of this "convergent" stuff. (That proof is here plus other proofs of it!)
Luckily, at least, this notion of convergents, even if it may not always be the best way to do it, lends itself to an easy way to check a solution to any such problem. Just find a bunch of the convergents - take as many as you need. If you somehow have multiple solutions, as you think with Ramanujan's radical, then you'll see the convergents get closer and closer to the "true" solution.
(How many convergents you need to find depends on the situation and how close your proposed solutions are. It might be immediately obvious after $10$ iterations, or might not be until $10,000,000$. This logic also relies on the assumption that there is only one solution to a given expression that is valid. Depending on the context, you might see cases where multiple solutions are valid but this "approaching by hand" method will only get you some of the solutions. This touches on the notion of "unstable" and "stable" solutions to dynamical systems - which I believe is the main context where such would pop up - but it's a bit overkill to discuss that for this post.)
So I will conclude by showing, in this way, that the solution is $3$ to Ramanujan's radical.
We begin with the radical itself:
$$sqrt{1+2sqrt{1+3sqrt{1+4sqrt{1+cdots}}}}=3$$
Let us begin by getting a series of convergents:
$$sqrt{1} ;;,;; sqrt{1 + 2sqrt{1}} ;;,;; sqrt{1 + 2sqrt{1 + 3sqrt{1}}} ;;,;;$$
Because the $sqrt{1}$ isn't necessary, we just let it be $1$.
$$1 ;;,;; sqrt{1 + 2} ;;,;; sqrt{1 + 2sqrt{1 + 3}} ;;,;;$$
Okay so ... where to go from here? Honestly, my initial temptation was to just use a MATLAB script and evaluate it, but I can't think of even a recursive closed form for this that would be nice enough. So in any event, we just have to go by "hand" (and by hand I mean WolframAlpha). Let $C(n)$ be the $n^{th}$ convergent. Then
- $C(1) = 1$
- $C(2) approx 1.732$
- $C(3) approx 2.236$
- $C(4) approx 2.560$
- $C(5) approx 2.755$
- $C(6) approx 2.867$
- $C(7) approx 2.929$
- $C(8) approx 2.963$
- $C(9) approx 2.981$
- $C(10) approx 2.990$
To skip a few values because at this point the changes get minimal, I used a macro to make a quick code for $C(50)$ so I could put it into Microsoft Excel and got the approximate result
$$C(50) approx 2.999 ; 999 ; 999 ; 999 ; 99$$
So while not the most rigorous result, we can at least on an intuitive level feel like the convergents from Ramanujan's radical converge to $3$, not $4$ or any other number. Neglecting that this is not an ironclad proof of the convergence, at least intuitively then we can feel like
$$3 = sqrt{1+2sqrt{1+3sqrt{1+4sqrt{1+cdots}}}}$$
Whew! Hopefully that lengthy post was helpful to you!
A late footnote, but Mathologer on YouTube did a video on this very topic, so his video would give a decent summary of all this stuff as well. Here's a link.
$endgroup$
Introduction:
The issue is what "..." really "represents."
Typically we use it as a sort of shorthand, as if to say "look, I can't write infinitely many things down, just assume that the obvious pattern holds and goes on infinitely."
This idea holds for all sorts of things - nested radicals, infinite sums, continued fractions, infinite sequences, etc.
On Infinite Sums:
A simple example: the sum of the reciprocals of squares:
$$1 + frac{1}{4} + frac{1}{9} + frac{1}{16} + ...$$
This is a well known summation. It is the Riemann zeta function $zeta(s)$ at $s=2$, and is known to evaluate to $pi^2/6$ (proved by Euler and known as the Basel problem).
Another, easier-to-handle summation is the geometric sum
$$1 + frac 1 2 + frac 1 4 + frac 1 8 + ...$$
This is a geometric series where the ratio is $1/2$ - each summand is half the previous one. We know, too, that this evaluates to $2$.
Another geometric series you might see in proofs that $0.999... = 1$ is
$$9 left( frac{1}{10} + frac{1}{100} + frac{1}{1,000} + frac{1}{10,000} + ... right)$$
which equals $1$. In fact, any infinite geometric series, with first term $a$ and ratio $|r|<1$ can be evaluated by
$$sum_{n=0}^infty ar^n = frac{a}{1-r}$$
So a question arises - ignoring these "obvious" results (depending on your amount of mathematical knowledge), how would we know these converge to the given values? What, exactly, does it mean for a summation to converge to a number or equal a number? For finite sums this is no issue - if nothing else, we could add up each number manually, but we can't just add up every number from a set of infinitely-many numbers.
Well, one could argue by common sense that, if the sequence converges to some number, the more and more terms you add up, the closer they'll get to that number.
So we obtain one definition for the convergence of an infinite sum. Consider a sequence where the $n^{th}$ term is defined by the sum of the first $n$ terms in the sequence. To introduce some symbols, suppose we're trying to find the sum
$$sum_{k=1}^infty x_k = x_1 + x_2 + x_3 + x_4 + ...$$
for whatever these $x_i$'s are. Then define these so-called "partial sums" of this by a function $S(n)$:
$$S(n) = sum_{k=1}^n x_k = x_1 + x_2 + ... + x_n$$
Then we get a sequence of sums:
$$S(1), S(2), S(3), ...$$
or equivalently
$$x_1 ;;,;; x_1 + x_2;;,;; x_1 + x_2 + x_3;;,;; ...$$
Then we ask: what does $S(n)$ approach as $n$ grows without bound, if anything at all? (In calculus, we call this "the limit of the partial sums $S(n)$ as $n$ approaches infinity.")
For the case of our first geometric sum, we immediately see the sequence of partial sums
$$1, frac{3}{2}, frac{7}{4}, frac{15}{8},...$$
Clearly, this suggests a pattern - and if you want to, you can go ahead and prove it, I won't do so here for brevity's sake. The pattern is that the $n^{th}$ term of the sequence is
$$S(n) = frac{2^{(n+1)}-1}{2^{n}}$$
We can then easily consider the limit of these partial sums:
$$lim_{ntoinfty} S(n) = lim_{ntoinfty} frac{2^{(n+1)}-1}{2^{n}} = lim_{ntoinfty} frac{2^{(n+1)}}{2^{n}} - frac {1}{2^{n}} = lim_{ntoinfty} 2 - lim_{ntoinfty} frac{1}{2^{n}}$$
Obviously, $1/2^{n} to 0$ as $n$ grows without bound, and $2$ is not affected by $n$, so we conclude
$$lim_{ntoinfty} S(n) = lim_{ntoinfty} 2 - lim_{ntoinfty} frac{1}{2^n} = 2 - 0 = 2$$
And thus we say
$$sum_{k=0}^infty left(frac 1 2 right)^k = 1 + frac 1 2 + frac 1 4 + frac 1 8 + ... = 2$$
because the partial sums approach $2$.
On Continued Fractions:
That was a simple, "first" sort of example, but mathematicians essentially do the same thing in other contexts. I want to touch on one more such context before we deal with the radical case, just to nail that point home.
In this case, it will be with continued fractions. One of the simpler such fractions is the one for $1$:
$$1 = frac{1}{2-frac{1}{2-frac{1}{2-frac{1}{...}}}}$$
As usual, the "..." denotes that this continues forever. But what it does it mean for this infinite expression to equal $1$?
For this, we consider a more general analogue of the "partial sum" from before - a "convergent." We cut up the sequence at logical finite points, whatever those points being depending on the context. Then if the sequence of the convergents approaches a limit, we say they're equal.
What are the convergents for a continued fraction? By convention, we cut off just before the start of the next fraction. That is, in the continued fraction for $1$, we cut off at the $n^{th} ; 2$ for the $n^{th}$ convergent, and ignore what follows. So we get the sequence of convergents
$$frac{1}{2} , frac{1}{2-frac{1}{2}}, frac{1}{2-frac{1}{2-frac{1}{2}}},...$$
Working out the numbers, we find the sequence to be
$$frac{1}{2},frac{2}{3},frac{3}{4},...$$
Again, we see a pattern! The $n^{th}$ term of the sequence is clearly of the form
$$frac{n-1}{n}$$
Let $C(n)$ be a function denoting the $n^{th}$ convergent. Then $C(1)=1/2,$ $C(2) = 2/3,$ $C(n)=(n-1)/n,$ and so on. So like before we consider the infinite limit:
$$lim_{ntoinfty} C(n) = lim_{ntoinfty} frac{n-1}{n} = lim_{ntoinfty} 1 - frac 1 n = lim_{ntoinfty} 1 - lim_{ntoinfty} frac 1 n = 1 - 0 = 1$$
Thus we can conclude that the continued fraction equals $1$, because its sequence of convergents equals $1$!
On Infinite Radicals:
So now, we touch on infinite nested radicals. They're messier to deal with but doable.
One of the simpler examples of such radicals to contend with is
$$2 = sqrt{2 +sqrt{2 +sqrt{2 +sqrt{2 +sqrt{2 +...}}}}}$$
As with the previous two cases we see an infinite expression. We instinctively conclude by now: to logically define a limit for this expression - to assign it a value provided it even exists - we need to chop this up at finite points, defining a sequence of convergents $C(n)$, and then find $C(n)$ as $ntoinfty$.
Nested radicals are a lot messier than the previous, but we manage.
So first let the sequence of convergents be given by cutting off everything after the $n^{th} ; 2$ in the expression. Thus we get the sequence
$$sqrt 2 ;;,;; sqrt{2 + sqrt{2}};;,;; sqrt{2+sqrt{2+sqrt{2}}};;,;; sqrt{2+sqrt{2+sqrt{2+sqrt{2}}}}$$
Okay this isn't particularly nice already, but apparently there does exist, shockingly enough, a closed-form explicit expression for $C(n)$: (from: S. Zimmerman, C. Ho)
$$C(n) = 2cosleft(frac{pi}{2^{n+1}}right)$$
(I had to find that expression by Googling, I honestly didn't know that offhand. It can be proved by induction, as touched on in this MSE question.)
So luckily, then, we can find the limit of $C(n)$:
$$lim_{ntoinfty} C(n) = lim_{ntoinfty} 2cosleft(frac{pi}{2^{n+1}}right)$$
It is probably obvious that the argument of the cosine function approaches $0$ as $n$ grows without bound, and thus
$$lim_{ntoinfty} C(n) = lim_{ntoinfty} 2cosleft(frac{pi}{2^{n+1}}right) = 2cos(0) = 2cdot 1 = 2$$
Thus, since its convergents approach $2$, we can conclude that
$$2 = sqrt{2 +sqrt{2 +sqrt{2 +sqrt{2 +sqrt{2 +...}}}}}$$
A Lengthy Conclusion:
So, in short, how do we evaluate an infinite expression, be it radical, continued fraction, sum, or otherwise?
We begin by truncating the expression at convenient finite places, creating a series of convergents, generalizations of the "partial sums" introduced in calculus. We then try to get a closed form or some other usable expression for the convergents $C(n)$, and consider the value as $ntoinfty$. If it converges to some value, we say that the expression is in fact equal to that value. If it doesn't, then the expression doesn't converge to any value.
This doesn't mean each expression is "nice." Radical expressions in particular, in my experience, tend to be nasty as all hell, and I'm lucky I found that one closed form expression for the particularly easy radical I chose.
This doesn't mean that other methods cannot be used to find the values, so long as there's some sort of logical justification for said method. For example, there is a justification for the formula for an infinite (and finite) geometric sum. We might have to circumvent the notion of partial sums entirely, or at least it might be convenient to do so. For example, with the Basel problem, Euler's proof focused on Maclaurin series, and none of this "convergent" stuff. (That proof is here plus other proofs of it!)
Luckily, at least, this notion of convergents, even if it may not always be the best way to do it, lends itself to an easy way to check a solution to any such problem. Just find a bunch of the convergents - take as many as you need. If you somehow have multiple solutions, as you think with Ramanujan's radical, then you'll see the convergents get closer and closer to the "true" solution.
(How many convergents you need to find depends on the situation and how close your proposed solutions are. It might be immediately obvious after $10$ iterations, or might not be until $10,000,000$. This logic also relies on the assumption that there is only one solution to a given expression that is valid. Depending on the context, you might see cases where multiple solutions are valid but this "approaching by hand" method will only get you some of the solutions. This touches on the notion of "unstable" and "stable" solutions to dynamical systems - which I believe is the main context where such would pop up - but it's a bit overkill to discuss that for this post.)
So I will conclude by showing, in this way, that the solution is $3$ to Ramanujan's radical.
We begin with the radical itself:
$$sqrt{1+2sqrt{1+3sqrt{1+4sqrt{1+cdots}}}}=3$$
Let us begin by getting a series of convergents:
$$sqrt{1} ;;,;; sqrt{1 + 2sqrt{1}} ;;,;; sqrt{1 + 2sqrt{1 + 3sqrt{1}}} ;;,;;$$
Because the $sqrt{1}$ isn't necessary, we just let it be $1$.
$$1 ;;,;; sqrt{1 + 2} ;;,;; sqrt{1 + 2sqrt{1 + 3}} ;;,;;$$
Okay so ... where to go from here? Honestly, my initial temptation was to just use a MATLAB script and evaluate it, but I can't think of even a recursive closed form for this that would be nice enough. So in any event, we just have to go by "hand" (and by hand I mean WolframAlpha). Let $C(n)$ be the $n^{th}$ convergent. Then
- $C(1) = 1$
- $C(2) approx 1.732$
- $C(3) approx 2.236$
- $C(4) approx 2.560$
- $C(5) approx 2.755$
- $C(6) approx 2.867$
- $C(7) approx 2.929$
- $C(8) approx 2.963$
- $C(9) approx 2.981$
- $C(10) approx 2.990$
To skip a few values because at this point the changes get minimal, I used a macro to make a quick code for $C(50)$ so I could put it into Microsoft Excel and got the approximate result
$$C(50) approx 2.999 ; 999 ; 999 ; 999 ; 99$$
So while not the most rigorous result, we can at least on an intuitive level feel like the convergents from Ramanujan's radical converge to $3$, not $4$ or any other number. Neglecting that this is not an ironclad proof of the convergence, at least intuitively then we can feel like
$$3 = sqrt{1+2sqrt{1+3sqrt{1+4sqrt{1+cdots}}}}$$
Whew! Hopefully that lengthy post was helpful to you!
A late footnote, but Mathologer on YouTube did a video on this very topic, so his video would give a decent summary of all this stuff as well. Here's a link.
edited 10 hours ago


ronorono
31
31
answered yesterday


Eevee TrainerEevee Trainer
6,51311237
6,51311237
3
$begingroup$
On Mathematica you can dof[x_, n_] := Sqrt[1 + n * x]; ram[x0_, n_] := Fold[f, Sqrt[x0], Reverse @ Range[2, n]];
The results suggest that the radical approaches 3 regardless of the innermost initial value.
$endgroup$
– lastresort
yesterday
add a comment |
3
$begingroup$
On Mathematica you can dof[x_, n_] := Sqrt[1 + n * x]; ram[x0_, n_] := Fold[f, Sqrt[x0], Reverse @ Range[2, n]];
The results suggest that the radical approaches 3 regardless of the innermost initial value.
$endgroup$
– lastresort
yesterday
3
3
$begingroup$
On Mathematica you can do
f[x_, n_] := Sqrt[1 + n * x]; ram[x0_, n_] := Fold[f, Sqrt[x0], Reverse @ Range[2, n]];
The results suggest that the radical approaches 3 regardless of the innermost initial value.$endgroup$
– lastresort
yesterday
$begingroup$
On Mathematica you can do
f[x_, n_] := Sqrt[1 + n * x]; ram[x0_, n_] := Fold[f, Sqrt[x0], Reverse @ Range[2, n]];
The results suggest that the radical approaches 3 regardless of the innermost initial value.$endgroup$
– lastresort
yesterday
add a comment |
$begingroup$
The user @Eevee Trainer provided a nice explanation on how we define infinite nested radical in terms of limit of finite nested radical which should be insensitive of the starting point. For full generality in this regard, we can consider the convergence of the following finite nested radical
$$ sqrt{1 + 2 sqrt{1 + 3 sqrt{ 1 + cdots n sqrt{1 + (n+1) a_n}}}} tag{*} $$
for a given sequence $(a_n)$ of non-negative real numbers. In this answer, I will prove the convergence of $text{(*)}$ to $3$ under a mild condition. My solution will involve some preliminary knowledge on analysis.
Setting. We consider the map $Phi$, defined on the space of all functions from $[0,infty)$ to $[0, infty)$, which is given by
$$ Phi [f](x) = sqrt{1 + xf(x+1)}. $$
Let us check how $Phi$ is related to our problem. The idea is to apply the trick of computing Ramanujan's infinite nested radical not to a single number, but rather to a function. Here, we choose $F(x) = x+1$. Since
$$ F(x) = 1+x = sqrt{1+x(x+2)} = sqrt{1 + xF(x+1)} = Phi[F](x), $$
it follows that we can iterated $Phi$ several times to obtain
$$ F(x) = Phi^{circ n}[F](x) = sqrt{1 + xsqrt{1 + (x+1)sqrt{1 + cdots (x+n-1)sqrt{(x+n+1)^2}}}}, $$
where $Phi^{circ n} = Phi circ cdots circ Phi$ is the $n$-fold function composition of $Phi$. Of course, the original radical corresponds to the case $x = 2$. This already proves that $Phi^{circ n}[F](x)$ converges to $F(x)$ as $ntoinfty$.
On the other hand, infinite nested radicals do not have any designated value to start with, and so, the above computation is far from satisfactory when it comes to defining infinite nested radical. Thus, a form of robustness of the convergence is required. In this regard, @Eevee Trainer investigated the convergence of
$$Phi^{circ n}[1](2) = sqrt{1 + 2sqrt{1 + 3sqrt{1 + cdots (n+1)sqrt{1}}}}, $$
and confirmed numerically that this still tends to $F(2) = 3$ as $n$ grows. Of course, it will be ideal if we can verify the same conclusion for other choices starting points and using rigorous argument.
Proof. One nice thing about $Phi$ is that it enjoys monotone, i.e., if $f leq g$, then $Phi [f] leq Phi [g]$. From this, it is easy to establish the following observation.
Lemma 1. For any $f geq 0$, we have $liminf_{ntoinfty} Phi^{circ n}[f](x) geq x+1$.
Proof. We inductively apply the monotonicity of $Phi$ to find that
$$Phi^{circ (n+1)} [0](x) geq x^{1-2^{-n}} qquad text{and} qquad Phi^{circ n}[x](x) geq x + 1 - 2^{-n}. $$
So, for any integer $m geq 0$,
begin{align*}
liminf_{ntoinfty} Phi^{circ n}[f](x)
&geq liminf_{ntoinfty} Phi^{circ m}[Phi^{circ (n+1)}[0]](x)
geq liminf_{ntoinfty} Phi^{circ m}[x^{1-2^{-(n-1)}}](x) \
&= Phi^{circ m}[x](x)
geq x + 1 - 2^{-m},
end{align*}
and letting $m to infty$ proves Lemma 1 as required.
Lemma 2. If $limsup_{xtoinfty} frac{log log max{e, f(x)}}{x} < log 2$, then $limsup_{ntoinfty} Phi^{circ n}[f](x) leq x+1$.
Proof. By the assumption, there exists $C > 1$ and $alpha in [0, 2)$ such that $f(x) leq C e^{alpha^x} (x + 1)$. Again, applying monotonicity of $Phi$, we check that $Phi^{circ n}[f](x) leq C^{2^{-n}} e^{(alpha/2)^n alpha^x} (x+1)$ holds. This is certainly true if $n = 0$. Moreover, assuming that this is true for $n$,
begin{align*}
Phi^{circ (n+1)}[f](x)
&leq Phileft[C^{2^{-n}} e^{(alpha/2)^n alpha^x} (x+1)right](x) \
&= left( 1 + x C^{2^{-n}} e^{(alpha/2)^n alpha^{x+1}} (x+2) right)^{1/2} \
&leq C^{2^{-n-1}} e^{(alpha/2)^{n+1} alpha^{x}} (x+1).
end{align*}
Now letting $n to infty$ proves the desired result.
Corollary. If $a_n geq 0$ satisfies $limsup_{ntoinfty} frac{loglog max{e, a_n}}{n} < log 2$, then for any $x geq 0$,
$$ lim_{ntoinfty} sqrt{1 + x sqrt{1 + (x+1) sqrt{ 1 + cdots + (x+n-2) sqrt{1 + (x+n-1) a_n }}}} = x+1. $$
Proof. Apply Lemma 1 and 2 to the function $f$ which interpolates $(a_n)$, such as using piecewise linear interpolation.
The bound in Lemma 2 and Corollary is optimal. Indeed, consider the non-example in OP's question of expanding $4$ as in Ramanujan's infinite nested radical. So we define the sequence $a_n$ so as to satisfy
$$ sqrt{1 + 2 sqrt{1 + 3 sqrt{ 1 + cdots n sqrt{1 + (n+1) a_n}}}} = 4. $$
Its first 4 terms are given as follows.
$$ a_1 = frac{15}{2}, quad
a_2 = frac{221}{12}, quad
a_3 = frac{48697}{576}, quad
a_4 = frac{2371066033}{1658880}, quad cdots. $$
Then we can show that $frac{1}{n}log log a_n to log 2$ as $n to infty$.
$endgroup$
add a comment |
$begingroup$
The user @Eevee Trainer provided a nice explanation on how we define infinite nested radical in terms of limit of finite nested radical which should be insensitive of the starting point. For full generality in this regard, we can consider the convergence of the following finite nested radical
$$ sqrt{1 + 2 sqrt{1 + 3 sqrt{ 1 + cdots n sqrt{1 + (n+1) a_n}}}} tag{*} $$
for a given sequence $(a_n)$ of non-negative real numbers. In this answer, I will prove the convergence of $text{(*)}$ to $3$ under a mild condition. My solution will involve some preliminary knowledge on analysis.
Setting. We consider the map $Phi$, defined on the space of all functions from $[0,infty)$ to $[0, infty)$, which is given by
$$ Phi [f](x) = sqrt{1 + xf(x+1)}. $$
Let us check how $Phi$ is related to our problem. The idea is to apply the trick of computing Ramanujan's infinite nested radical not to a single number, but rather to a function. Here, we choose $F(x) = x+1$. Since
$$ F(x) = 1+x = sqrt{1+x(x+2)} = sqrt{1 + xF(x+1)} = Phi[F](x), $$
it follows that we can iterated $Phi$ several times to obtain
$$ F(x) = Phi^{circ n}[F](x) = sqrt{1 + xsqrt{1 + (x+1)sqrt{1 + cdots (x+n-1)sqrt{(x+n+1)^2}}}}, $$
where $Phi^{circ n} = Phi circ cdots circ Phi$ is the $n$-fold function composition of $Phi$. Of course, the original radical corresponds to the case $x = 2$. This already proves that $Phi^{circ n}[F](x)$ converges to $F(x)$ as $ntoinfty$.
On the other hand, infinite nested radicals do not have any designated value to start with, and so, the above computation is far from satisfactory when it comes to defining infinite nested radical. Thus, a form of robustness of the convergence is required. In this regard, @Eevee Trainer investigated the convergence of
$$Phi^{circ n}[1](2) = sqrt{1 + 2sqrt{1 + 3sqrt{1 + cdots (n+1)sqrt{1}}}}, $$
and confirmed numerically that this still tends to $F(2) = 3$ as $n$ grows. Of course, it will be ideal if we can verify the same conclusion for other choices starting points and using rigorous argument.
Proof. One nice thing about $Phi$ is that it enjoys monotone, i.e., if $f leq g$, then $Phi [f] leq Phi [g]$. From this, it is easy to establish the following observation.
Lemma 1. For any $f geq 0$, we have $liminf_{ntoinfty} Phi^{circ n}[f](x) geq x+1$.
Proof. We inductively apply the monotonicity of $Phi$ to find that
$$Phi^{circ (n+1)} [0](x) geq x^{1-2^{-n}} qquad text{and} qquad Phi^{circ n}[x](x) geq x + 1 - 2^{-n}. $$
So, for any integer $m geq 0$,
begin{align*}
liminf_{ntoinfty} Phi^{circ n}[f](x)
&geq liminf_{ntoinfty} Phi^{circ m}[Phi^{circ (n+1)}[0]](x)
geq liminf_{ntoinfty} Phi^{circ m}[x^{1-2^{-(n-1)}}](x) \
&= Phi^{circ m}[x](x)
geq x + 1 - 2^{-m},
end{align*}
and letting $m to infty$ proves Lemma 1 as required.
Lemma 2. If $limsup_{xtoinfty} frac{log log max{e, f(x)}}{x} < log 2$, then $limsup_{ntoinfty} Phi^{circ n}[f](x) leq x+1$.
Proof. By the assumption, there exists $C > 1$ and $alpha in [0, 2)$ such that $f(x) leq C e^{alpha^x} (x + 1)$. Again, applying monotonicity of $Phi$, we check that $Phi^{circ n}[f](x) leq C^{2^{-n}} e^{(alpha/2)^n alpha^x} (x+1)$ holds. This is certainly true if $n = 0$. Moreover, assuming that this is true for $n$,
begin{align*}
Phi^{circ (n+1)}[f](x)
&leq Phileft[C^{2^{-n}} e^{(alpha/2)^n alpha^x} (x+1)right](x) \
&= left( 1 + x C^{2^{-n}} e^{(alpha/2)^n alpha^{x+1}} (x+2) right)^{1/2} \
&leq C^{2^{-n-1}} e^{(alpha/2)^{n+1} alpha^{x}} (x+1).
end{align*}
Now letting $n to infty$ proves the desired result.
Corollary. If $a_n geq 0$ satisfies $limsup_{ntoinfty} frac{loglog max{e, a_n}}{n} < log 2$, then for any $x geq 0$,
$$ lim_{ntoinfty} sqrt{1 + x sqrt{1 + (x+1) sqrt{ 1 + cdots + (x+n-2) sqrt{1 + (x+n-1) a_n }}}} = x+1. $$
Proof. Apply Lemma 1 and 2 to the function $f$ which interpolates $(a_n)$, such as using piecewise linear interpolation.
The bound in Lemma 2 and Corollary is optimal. Indeed, consider the non-example in OP's question of expanding $4$ as in Ramanujan's infinite nested radical. So we define the sequence $a_n$ so as to satisfy
$$ sqrt{1 + 2 sqrt{1 + 3 sqrt{ 1 + cdots n sqrt{1 + (n+1) a_n}}}} = 4. $$
Its first 4 terms are given as follows.
$$ a_1 = frac{15}{2}, quad
a_2 = frac{221}{12}, quad
a_3 = frac{48697}{576}, quad
a_4 = frac{2371066033}{1658880}, quad cdots. $$
Then we can show that $frac{1}{n}log log a_n to log 2$ as $n to infty$.
$endgroup$
add a comment |
$begingroup$
The user @Eevee Trainer provided a nice explanation on how we define infinite nested radical in terms of limit of finite nested radical which should be insensitive of the starting point. For full generality in this regard, we can consider the convergence of the following finite nested radical
$$ sqrt{1 + 2 sqrt{1 + 3 sqrt{ 1 + cdots n sqrt{1 + (n+1) a_n}}}} tag{*} $$
for a given sequence $(a_n)$ of non-negative real numbers. In this answer, I will prove the convergence of $text{(*)}$ to $3$ under a mild condition. My solution will involve some preliminary knowledge on analysis.
Setting. We consider the map $Phi$, defined on the space of all functions from $[0,infty)$ to $[0, infty)$, which is given by
$$ Phi [f](x) = sqrt{1 + xf(x+1)}. $$
Let us check how $Phi$ is related to our problem. The idea is to apply the trick of computing Ramanujan's infinite nested radical not to a single number, but rather to a function. Here, we choose $F(x) = x+1$. Since
$$ F(x) = 1+x = sqrt{1+x(x+2)} = sqrt{1 + xF(x+1)} = Phi[F](x), $$
it follows that we can iterated $Phi$ several times to obtain
$$ F(x) = Phi^{circ n}[F](x) = sqrt{1 + xsqrt{1 + (x+1)sqrt{1 + cdots (x+n-1)sqrt{(x+n+1)^2}}}}, $$
where $Phi^{circ n} = Phi circ cdots circ Phi$ is the $n$-fold function composition of $Phi$. Of course, the original radical corresponds to the case $x = 2$. This already proves that $Phi^{circ n}[F](x)$ converges to $F(x)$ as $ntoinfty$.
On the other hand, infinite nested radicals do not have any designated value to start with, and so, the above computation is far from satisfactory when it comes to defining infinite nested radical. Thus, a form of robustness of the convergence is required. In this regard, @Eevee Trainer investigated the convergence of
$$Phi^{circ n}[1](2) = sqrt{1 + 2sqrt{1 + 3sqrt{1 + cdots (n+1)sqrt{1}}}}, $$
and confirmed numerically that this still tends to $F(2) = 3$ as $n$ grows. Of course, it will be ideal if we can verify the same conclusion for other choices starting points and using rigorous argument.
Proof. One nice thing about $Phi$ is that it enjoys monotone, i.e., if $f leq g$, then $Phi [f] leq Phi [g]$. From this, it is easy to establish the following observation.
Lemma 1. For any $f geq 0$, we have $liminf_{ntoinfty} Phi^{circ n}[f](x) geq x+1$.
Proof. We inductively apply the monotonicity of $Phi$ to find that
$$Phi^{circ (n+1)} [0](x) geq x^{1-2^{-n}} qquad text{and} qquad Phi^{circ n}[x](x) geq x + 1 - 2^{-n}. $$
So, for any integer $m geq 0$,
begin{align*}
liminf_{ntoinfty} Phi^{circ n}[f](x)
&geq liminf_{ntoinfty} Phi^{circ m}[Phi^{circ (n+1)}[0]](x)
geq liminf_{ntoinfty} Phi^{circ m}[x^{1-2^{-(n-1)}}](x) \
&= Phi^{circ m}[x](x)
geq x + 1 - 2^{-m},
end{align*}
and letting $m to infty$ proves Lemma 1 as required.
Lemma 2. If $limsup_{xtoinfty} frac{log log max{e, f(x)}}{x} < log 2$, then $limsup_{ntoinfty} Phi^{circ n}[f](x) leq x+1$.
Proof. By the assumption, there exists $C > 1$ and $alpha in [0, 2)$ such that $f(x) leq C e^{alpha^x} (x + 1)$. Again, applying monotonicity of $Phi$, we check that $Phi^{circ n}[f](x) leq C^{2^{-n}} e^{(alpha/2)^n alpha^x} (x+1)$ holds. This is certainly true if $n = 0$. Moreover, assuming that this is true for $n$,
begin{align*}
Phi^{circ (n+1)}[f](x)
&leq Phileft[C^{2^{-n}} e^{(alpha/2)^n alpha^x} (x+1)right](x) \
&= left( 1 + x C^{2^{-n}} e^{(alpha/2)^n alpha^{x+1}} (x+2) right)^{1/2} \
&leq C^{2^{-n-1}} e^{(alpha/2)^{n+1} alpha^{x}} (x+1).
end{align*}
Now letting $n to infty$ proves the desired result.
Corollary. If $a_n geq 0$ satisfies $limsup_{ntoinfty} frac{loglog max{e, a_n}}{n} < log 2$, then for any $x geq 0$,
$$ lim_{ntoinfty} sqrt{1 + x sqrt{1 + (x+1) sqrt{ 1 + cdots + (x+n-2) sqrt{1 + (x+n-1) a_n }}}} = x+1. $$
Proof. Apply Lemma 1 and 2 to the function $f$ which interpolates $(a_n)$, such as using piecewise linear interpolation.
The bound in Lemma 2 and Corollary is optimal. Indeed, consider the non-example in OP's question of expanding $4$ as in Ramanujan's infinite nested radical. So we define the sequence $a_n$ so as to satisfy
$$ sqrt{1 + 2 sqrt{1 + 3 sqrt{ 1 + cdots n sqrt{1 + (n+1) a_n}}}} = 4. $$
Its first 4 terms are given as follows.
$$ a_1 = frac{15}{2}, quad
a_2 = frac{221}{12}, quad
a_3 = frac{48697}{576}, quad
a_4 = frac{2371066033}{1658880}, quad cdots. $$
Then we can show that $frac{1}{n}log log a_n to log 2$ as $n to infty$.
$endgroup$
The user @Eevee Trainer provided a nice explanation on how we define infinite nested radical in terms of limit of finite nested radical which should be insensitive of the starting point. For full generality in this regard, we can consider the convergence of the following finite nested radical
$$ sqrt{1 + 2 sqrt{1 + 3 sqrt{ 1 + cdots n sqrt{1 + (n+1) a_n}}}} tag{*} $$
for a given sequence $(a_n)$ of non-negative real numbers. In this answer, I will prove the convergence of $text{(*)}$ to $3$ under a mild condition. My solution will involve some preliminary knowledge on analysis.
Setting. We consider the map $Phi$, defined on the space of all functions from $[0,infty)$ to $[0, infty)$, which is given by
$$ Phi [f](x) = sqrt{1 + xf(x+1)}. $$
Let us check how $Phi$ is related to our problem. The idea is to apply the trick of computing Ramanujan's infinite nested radical not to a single number, but rather to a function. Here, we choose $F(x) = x+1$. Since
$$ F(x) = 1+x = sqrt{1+x(x+2)} = sqrt{1 + xF(x+1)} = Phi[F](x), $$
it follows that we can iterated $Phi$ several times to obtain
$$ F(x) = Phi^{circ n}[F](x) = sqrt{1 + xsqrt{1 + (x+1)sqrt{1 + cdots (x+n-1)sqrt{(x+n+1)^2}}}}, $$
where $Phi^{circ n} = Phi circ cdots circ Phi$ is the $n$-fold function composition of $Phi$. Of course, the original radical corresponds to the case $x = 2$. This already proves that $Phi^{circ n}[F](x)$ converges to $F(x)$ as $ntoinfty$.
On the other hand, infinite nested radicals do not have any designated value to start with, and so, the above computation is far from satisfactory when it comes to defining infinite nested radical. Thus, a form of robustness of the convergence is required. In this regard, @Eevee Trainer investigated the convergence of
$$Phi^{circ n}[1](2) = sqrt{1 + 2sqrt{1 + 3sqrt{1 + cdots (n+1)sqrt{1}}}}, $$
and confirmed numerically that this still tends to $F(2) = 3$ as $n$ grows. Of course, it will be ideal if we can verify the same conclusion for other choices starting points and using rigorous argument.
Proof. One nice thing about $Phi$ is that it enjoys monotone, i.e., if $f leq g$, then $Phi [f] leq Phi [g]$. From this, it is easy to establish the following observation.
Lemma 1. For any $f geq 0$, we have $liminf_{ntoinfty} Phi^{circ n}[f](x) geq x+1$.
Proof. We inductively apply the monotonicity of $Phi$ to find that
$$Phi^{circ (n+1)} [0](x) geq x^{1-2^{-n}} qquad text{and} qquad Phi^{circ n}[x](x) geq x + 1 - 2^{-n}. $$
So, for any integer $m geq 0$,
begin{align*}
liminf_{ntoinfty} Phi^{circ n}[f](x)
&geq liminf_{ntoinfty} Phi^{circ m}[Phi^{circ (n+1)}[0]](x)
geq liminf_{ntoinfty} Phi^{circ m}[x^{1-2^{-(n-1)}}](x) \
&= Phi^{circ m}[x](x)
geq x + 1 - 2^{-m},
end{align*}
and letting $m to infty$ proves Lemma 1 as required.
Lemma 2. If $limsup_{xtoinfty} frac{log log max{e, f(x)}}{x} < log 2$, then $limsup_{ntoinfty} Phi^{circ n}[f](x) leq x+1$.
Proof. By the assumption, there exists $C > 1$ and $alpha in [0, 2)$ such that $f(x) leq C e^{alpha^x} (x + 1)$. Again, applying monotonicity of $Phi$, we check that $Phi^{circ n}[f](x) leq C^{2^{-n}} e^{(alpha/2)^n alpha^x} (x+1)$ holds. This is certainly true if $n = 0$. Moreover, assuming that this is true for $n$,
begin{align*}
Phi^{circ (n+1)}[f](x)
&leq Phileft[C^{2^{-n}} e^{(alpha/2)^n alpha^x} (x+1)right](x) \
&= left( 1 + x C^{2^{-n}} e^{(alpha/2)^n alpha^{x+1}} (x+2) right)^{1/2} \
&leq C^{2^{-n-1}} e^{(alpha/2)^{n+1} alpha^{x}} (x+1).
end{align*}
Now letting $n to infty$ proves the desired result.
Corollary. If $a_n geq 0$ satisfies $limsup_{ntoinfty} frac{loglog max{e, a_n}}{n} < log 2$, then for any $x geq 0$,
$$ lim_{ntoinfty} sqrt{1 + x sqrt{1 + (x+1) sqrt{ 1 + cdots + (x+n-2) sqrt{1 + (x+n-1) a_n }}}} = x+1. $$
Proof. Apply Lemma 1 and 2 to the function $f$ which interpolates $(a_n)$, such as using piecewise linear interpolation.
The bound in Lemma 2 and Corollary is optimal. Indeed, consider the non-example in OP's question of expanding $4$ as in Ramanujan's infinite nested radical. So we define the sequence $a_n$ so as to satisfy
$$ sqrt{1 + 2 sqrt{1 + 3 sqrt{ 1 + cdots n sqrt{1 + (n+1) a_n}}}} = 4. $$
Its first 4 terms are given as follows.
$$ a_1 = frac{15}{2}, quad
a_2 = frac{221}{12}, quad
a_3 = frac{48697}{576}, quad
a_4 = frac{2371066033}{1658880}, quad cdots. $$
Then we can show that $frac{1}{n}log log a_n to log 2$ as $n to infty$.
edited 16 hours ago
answered 17 hours ago


Sangchul LeeSangchul Lee
94.3k12169274
94.3k12169274
add a comment |
add a comment |
$begingroup$
As others have said, the rigorous definition of an infinite expression comes from the limit of a sequence of finite terms. The terms need to be well-defined, but in practice, we just try to make sure the pattern is clear from context.
Now let's see what goes wrong with your other example. You wrote:
$$4 = sqrt{16}=sqrt{1+2sqrt{56.25}}=sqrt{1+2sqrt{1+3sqrt{frac{48841}{144}}}}=...=sqrt{1+2sqrt{1+3sqrt{1+4sqrt{1+cdots}}}}$$
The problem is that each term in the sequence (e.g. if we stop at $4$) fails to include an "extra" amount (and this amount is not going to zero). So if we look at the partial sums, we see they won't converge to $4$ unless we include the extra amounts we keep pushing to the right. It's the same logical mistake as taking
begin{align*}
2 &= 1 + 1 \
&= frac{1}{2} + frac{1}{2} + 1 \
&= frac{1}{2} + frac{1}{4} + frac{1}{4} + 1 \
&= frac{1}{2} + frac{1}{4} + frac{1}{8} + cdots + 1
end{align*}
And then saying, wait, the partial sums in the last line only converge to $1$ instead of $2$. But this is a mistake because we can't push the extra $1$ "infinitely far" right. Otherwise, the partial sum terms we write down will just look like $frac{1}{2} + frac{1}{4} + cdots$ and will never include the $1$. The same thing is happening (roughly) in your example.
$endgroup$
$begingroup$
However, the terms in the argument for the value being 3 are 16, 25, 36, ... also not converging to 0.
$endgroup$
– M.Herzkamp
23 hours ago
$begingroup$
@M.Herzkamp, I've edited to avoid that example. To look at the extra terms rigorously, you really have to subtract the entire term in the sequence from the total, which doesn't have a simple closed form in the square root examples.
$endgroup$
– usul
22 hours ago
add a comment |
$begingroup$
As others have said, the rigorous definition of an infinite expression comes from the limit of a sequence of finite terms. The terms need to be well-defined, but in practice, we just try to make sure the pattern is clear from context.
Now let's see what goes wrong with your other example. You wrote:
$$4 = sqrt{16}=sqrt{1+2sqrt{56.25}}=sqrt{1+2sqrt{1+3sqrt{frac{48841}{144}}}}=...=sqrt{1+2sqrt{1+3sqrt{1+4sqrt{1+cdots}}}}$$
The problem is that each term in the sequence (e.g. if we stop at $4$) fails to include an "extra" amount (and this amount is not going to zero). So if we look at the partial sums, we see they won't converge to $4$ unless we include the extra amounts we keep pushing to the right. It's the same logical mistake as taking
begin{align*}
2 &= 1 + 1 \
&= frac{1}{2} + frac{1}{2} + 1 \
&= frac{1}{2} + frac{1}{4} + frac{1}{4} + 1 \
&= frac{1}{2} + frac{1}{4} + frac{1}{8} + cdots + 1
end{align*}
And then saying, wait, the partial sums in the last line only converge to $1$ instead of $2$. But this is a mistake because we can't push the extra $1$ "infinitely far" right. Otherwise, the partial sum terms we write down will just look like $frac{1}{2} + frac{1}{4} + cdots$ and will never include the $1$. The same thing is happening (roughly) in your example.
$endgroup$
$begingroup$
However, the terms in the argument for the value being 3 are 16, 25, 36, ... also not converging to 0.
$endgroup$
– M.Herzkamp
23 hours ago
$begingroup$
@M.Herzkamp, I've edited to avoid that example. To look at the extra terms rigorously, you really have to subtract the entire term in the sequence from the total, which doesn't have a simple closed form in the square root examples.
$endgroup$
– usul
22 hours ago
add a comment |
$begingroup$
As others have said, the rigorous definition of an infinite expression comes from the limit of a sequence of finite terms. The terms need to be well-defined, but in practice, we just try to make sure the pattern is clear from context.
Now let's see what goes wrong with your other example. You wrote:
$$4 = sqrt{16}=sqrt{1+2sqrt{56.25}}=sqrt{1+2sqrt{1+3sqrt{frac{48841}{144}}}}=...=sqrt{1+2sqrt{1+3sqrt{1+4sqrt{1+cdots}}}}$$
The problem is that each term in the sequence (e.g. if we stop at $4$) fails to include an "extra" amount (and this amount is not going to zero). So if we look at the partial sums, we see they won't converge to $4$ unless we include the extra amounts we keep pushing to the right. It's the same logical mistake as taking
begin{align*}
2 &= 1 + 1 \
&= frac{1}{2} + frac{1}{2} + 1 \
&= frac{1}{2} + frac{1}{4} + frac{1}{4} + 1 \
&= frac{1}{2} + frac{1}{4} + frac{1}{8} + cdots + 1
end{align*}
And then saying, wait, the partial sums in the last line only converge to $1$ instead of $2$. But this is a mistake because we can't push the extra $1$ "infinitely far" right. Otherwise, the partial sum terms we write down will just look like $frac{1}{2} + frac{1}{4} + cdots$ and will never include the $1$. The same thing is happening (roughly) in your example.
$endgroup$
As others have said, the rigorous definition of an infinite expression comes from the limit of a sequence of finite terms. The terms need to be well-defined, but in practice, we just try to make sure the pattern is clear from context.
Now let's see what goes wrong with your other example. You wrote:
$$4 = sqrt{16}=sqrt{1+2sqrt{56.25}}=sqrt{1+2sqrt{1+3sqrt{frac{48841}{144}}}}=...=sqrt{1+2sqrt{1+3sqrt{1+4sqrt{1+cdots}}}}$$
The problem is that each term in the sequence (e.g. if we stop at $4$) fails to include an "extra" amount (and this amount is not going to zero). So if we look at the partial sums, we see they won't converge to $4$ unless we include the extra amounts we keep pushing to the right. It's the same logical mistake as taking
begin{align*}
2 &= 1 + 1 \
&= frac{1}{2} + frac{1}{2} + 1 \
&= frac{1}{2} + frac{1}{4} + frac{1}{4} + 1 \
&= frac{1}{2} + frac{1}{4} + frac{1}{8} + cdots + 1
end{align*}
And then saying, wait, the partial sums in the last line only converge to $1$ instead of $2$. But this is a mistake because we can't push the extra $1$ "infinitely far" right. Otherwise, the partial sum terms we write down will just look like $frac{1}{2} + frac{1}{4} + cdots$ and will never include the $1$. The same thing is happening (roughly) in your example.
edited 22 hours ago
answered yesterday
usulusul
1,6571422
1,6571422
$begingroup$
However, the terms in the argument for the value being 3 are 16, 25, 36, ... also not converging to 0.
$endgroup$
– M.Herzkamp
23 hours ago
$begingroup$
@M.Herzkamp, I've edited to avoid that example. To look at the extra terms rigorously, you really have to subtract the entire term in the sequence from the total, which doesn't have a simple closed form in the square root examples.
$endgroup$
– usul
22 hours ago
add a comment |
$begingroup$
However, the terms in the argument for the value being 3 are 16, 25, 36, ... also not converging to 0.
$endgroup$
– M.Herzkamp
23 hours ago
$begingroup$
@M.Herzkamp, I've edited to avoid that example. To look at the extra terms rigorously, you really have to subtract the entire term in the sequence from the total, which doesn't have a simple closed form in the square root examples.
$endgroup$
– usul
22 hours ago
$begingroup$
However, the terms in the argument for the value being 3 are 16, 25, 36, ... also not converging to 0.
$endgroup$
– M.Herzkamp
23 hours ago
$begingroup$
However, the terms in the argument for the value being 3 are 16, 25, 36, ... also not converging to 0.
$endgroup$
– M.Herzkamp
23 hours ago
$begingroup$
@M.Herzkamp, I've edited to avoid that example. To look at the extra terms rigorously, you really have to subtract the entire term in the sequence from the total, which doesn't have a simple closed form in the square root examples.
$endgroup$
– usul
22 hours ago
$begingroup$
@M.Herzkamp, I've edited to avoid that example. To look at the extra terms rigorously, you really have to subtract the entire term in the sequence from the total, which doesn't have a simple closed form in the square root examples.
$endgroup$
– usul
22 hours ago
add a comment |
$begingroup$
Your example doesn't quite capture the spirit of the Ramanujan expression: it arises from the succession of the integers, not from ad hoc computation to try to fit the expression to a value.
Let's make a sketch of an induction proof. Take the base case $n=3$:
$$sqrt{n^2}=3$$
and, for the inductive step, take the radical at the "deepest" level of the chain and apply
$$sqrt{k^2}=sqrt{1+(k-1)(k+1)}$$
$$=sqrt{1+(k-1)sqrt{(k+1)^2}}$$
this gives us exactly the sequence we need. This means we can take any $N>=3$ and construct the expression with $sqrt{N^2}$ in the deepest radical, and know that the equality with 3 has been preserved. (What does this tell us about the limit at infinity?)
For the 4 example, there's no analogous step to get from one term of the sequence to the next; you have to compute the term at the lowest level by working through the whole chain each time.
New contributor
ripkoops is a new contributor to this site. Take care in asking for clarification, commenting, and answering.
Check out our Code of Conduct.
$endgroup$
$begingroup$
Thanks for your help. However, gathering all the helps from the community so far, I would say I disagree with your points. In the case of '4' , we can definitely define a sequence of nested radicals(although the general term is super ugly) that is well-defined and converges to 4. The problem is the expression of the infinite nested radical alone is not telling us all the information. It is never properly defined by itself.
$endgroup$
– Anson NG
18 hours ago
$begingroup$
It true that your sequence converges to 4 (because that's how it's constructed), but in some sense it doesn't converge to the abstracted sequence in question here. Think about the expressionsqrt(1+2*sqrt(1+3*sqrt(1+...)))
. What's the additive contribution of the "..." part (i.e. the difference between this andsqrt(1+2*sqrt(1+3*sqrt(1)))
)? We should expect it to approach 0 as we go deeper into the chain if we want to arrive at a limit, but in your derivation you're squaring it at each term of the sequence, which offsets the reduction you'd see from it being within another square root.
$endgroup$
– ripkoops
9 hours ago
add a comment |
$begingroup$
Your example doesn't quite capture the spirit of the Ramanujan expression: it arises from the succession of the integers, not from ad hoc computation to try to fit the expression to a value.
Let's make a sketch of an induction proof. Take the base case $n=3$:
$$sqrt{n^2}=3$$
and, for the inductive step, take the radical at the "deepest" level of the chain and apply
$$sqrt{k^2}=sqrt{1+(k-1)(k+1)}$$
$$=sqrt{1+(k-1)sqrt{(k+1)^2}}$$
this gives us exactly the sequence we need. This means we can take any $N>=3$ and construct the expression with $sqrt{N^2}$ in the deepest radical, and know that the equality with 3 has been preserved. (What does this tell us about the limit at infinity?)
For the 4 example, there's no analogous step to get from one term of the sequence to the next; you have to compute the term at the lowest level by working through the whole chain each time.
New contributor
ripkoops is a new contributor to this site. Take care in asking for clarification, commenting, and answering.
Check out our Code of Conduct.
$endgroup$
$begingroup$
Thanks for your help. However, gathering all the helps from the community so far, I would say I disagree with your points. In the case of '4' , we can definitely define a sequence of nested radicals(although the general term is super ugly) that is well-defined and converges to 4. The problem is the expression of the infinite nested radical alone is not telling us all the information. It is never properly defined by itself.
$endgroup$
– Anson NG
18 hours ago
$begingroup$
It true that your sequence converges to 4 (because that's how it's constructed), but in some sense it doesn't converge to the abstracted sequence in question here. Think about the expressionsqrt(1+2*sqrt(1+3*sqrt(1+...)))
. What's the additive contribution of the "..." part (i.e. the difference between this andsqrt(1+2*sqrt(1+3*sqrt(1)))
)? We should expect it to approach 0 as we go deeper into the chain if we want to arrive at a limit, but in your derivation you're squaring it at each term of the sequence, which offsets the reduction you'd see from it being within another square root.
$endgroup$
– ripkoops
9 hours ago
add a comment |
$begingroup$
Your example doesn't quite capture the spirit of the Ramanujan expression: it arises from the succession of the integers, not from ad hoc computation to try to fit the expression to a value.
Let's make a sketch of an induction proof. Take the base case $n=3$:
$$sqrt{n^2}=3$$
and, for the inductive step, take the radical at the "deepest" level of the chain and apply
$$sqrt{k^2}=sqrt{1+(k-1)(k+1)}$$
$$=sqrt{1+(k-1)sqrt{(k+1)^2}}$$
this gives us exactly the sequence we need. This means we can take any $N>=3$ and construct the expression with $sqrt{N^2}$ in the deepest radical, and know that the equality with 3 has been preserved. (What does this tell us about the limit at infinity?)
For the 4 example, there's no analogous step to get from one term of the sequence to the next; you have to compute the term at the lowest level by working through the whole chain each time.
New contributor
ripkoops is a new contributor to this site. Take care in asking for clarification, commenting, and answering.
Check out our Code of Conduct.
$endgroup$
Your example doesn't quite capture the spirit of the Ramanujan expression: it arises from the succession of the integers, not from ad hoc computation to try to fit the expression to a value.
Let's make a sketch of an induction proof. Take the base case $n=3$:
$$sqrt{n^2}=3$$
and, for the inductive step, take the radical at the "deepest" level of the chain and apply
$$sqrt{k^2}=sqrt{1+(k-1)(k+1)}$$
$$=sqrt{1+(k-1)sqrt{(k+1)^2}}$$
this gives us exactly the sequence we need. This means we can take any $N>=3$ and construct the expression with $sqrt{N^2}$ in the deepest radical, and know that the equality with 3 has been preserved. (What does this tell us about the limit at infinity?)
For the 4 example, there's no analogous step to get from one term of the sequence to the next; you have to compute the term at the lowest level by working through the whole chain each time.
New contributor
ripkoops is a new contributor to this site. Take care in asking for clarification, commenting, and answering.
Check out our Code of Conduct.
New contributor
ripkoops is a new contributor to this site. Take care in asking for clarification, commenting, and answering.
Check out our Code of Conduct.
answered 20 hours ago
ripkoopsripkoops
312
312
New contributor
ripkoops is a new contributor to this site. Take care in asking for clarification, commenting, and answering.
Check out our Code of Conduct.
New contributor
ripkoops is a new contributor to this site. Take care in asking for clarification, commenting, and answering.
Check out our Code of Conduct.
ripkoops is a new contributor to this site. Take care in asking for clarification, commenting, and answering.
Check out our Code of Conduct.
$begingroup$
Thanks for your help. However, gathering all the helps from the community so far, I would say I disagree with your points. In the case of '4' , we can definitely define a sequence of nested radicals(although the general term is super ugly) that is well-defined and converges to 4. The problem is the expression of the infinite nested radical alone is not telling us all the information. It is never properly defined by itself.
$endgroup$
– Anson NG
18 hours ago
$begingroup$
It true that your sequence converges to 4 (because that's how it's constructed), but in some sense it doesn't converge to the abstracted sequence in question here. Think about the expressionsqrt(1+2*sqrt(1+3*sqrt(1+...)))
. What's the additive contribution of the "..." part (i.e. the difference between this andsqrt(1+2*sqrt(1+3*sqrt(1)))
)? We should expect it to approach 0 as we go deeper into the chain if we want to arrive at a limit, but in your derivation you're squaring it at each term of the sequence, which offsets the reduction you'd see from it being within another square root.
$endgroup$
– ripkoops
9 hours ago
add a comment |
$begingroup$
Thanks for your help. However, gathering all the helps from the community so far, I would say I disagree with your points. In the case of '4' , we can definitely define a sequence of nested radicals(although the general term is super ugly) that is well-defined and converges to 4. The problem is the expression of the infinite nested radical alone is not telling us all the information. It is never properly defined by itself.
$endgroup$
– Anson NG
18 hours ago
$begingroup$
It true that your sequence converges to 4 (because that's how it's constructed), but in some sense it doesn't converge to the abstracted sequence in question here. Think about the expressionsqrt(1+2*sqrt(1+3*sqrt(1+...)))
. What's the additive contribution of the "..." part (i.e. the difference between this andsqrt(1+2*sqrt(1+3*sqrt(1)))
)? We should expect it to approach 0 as we go deeper into the chain if we want to arrive at a limit, but in your derivation you're squaring it at each term of the sequence, which offsets the reduction you'd see from it being within another square root.
$endgroup$
– ripkoops
9 hours ago
$begingroup$
Thanks for your help. However, gathering all the helps from the community so far, I would say I disagree with your points. In the case of '4' , we can definitely define a sequence of nested radicals(although the general term is super ugly) that is well-defined and converges to 4. The problem is the expression of the infinite nested radical alone is not telling us all the information. It is never properly defined by itself.
$endgroup$
– Anson NG
18 hours ago
$begingroup$
Thanks for your help. However, gathering all the helps from the community so far, I would say I disagree with your points. In the case of '4' , we can definitely define a sequence of nested radicals(although the general term is super ugly) that is well-defined and converges to 4. The problem is the expression of the infinite nested radical alone is not telling us all the information. It is never properly defined by itself.
$endgroup$
– Anson NG
18 hours ago
$begingroup$
It true that your sequence converges to 4 (because that's how it's constructed), but in some sense it doesn't converge to the abstracted sequence in question here. Think about the expression
sqrt(1+2*sqrt(1+3*sqrt(1+...)))
. What's the additive contribution of the "..." part (i.e. the difference between this and sqrt(1+2*sqrt(1+3*sqrt(1)))
)? We should expect it to approach 0 as we go deeper into the chain if we want to arrive at a limit, but in your derivation you're squaring it at each term of the sequence, which offsets the reduction you'd see from it being within another square root.$endgroup$
– ripkoops
9 hours ago
$begingroup$
It true that your sequence converges to 4 (because that's how it's constructed), but in some sense it doesn't converge to the abstracted sequence in question here. Think about the expression
sqrt(1+2*sqrt(1+3*sqrt(1+...)))
. What's the additive contribution of the "..." part (i.e. the difference between this and sqrt(1+2*sqrt(1+3*sqrt(1)))
)? We should expect it to approach 0 as we go deeper into the chain if we want to arrive at a limit, but in your derivation you're squaring it at each term of the sequence, which offsets the reduction you'd see from it being within another square root.$endgroup$
– ripkoops
9 hours ago
add a comment |
$begingroup$
$4=sqrt{16}=sqrt{1+3sqrt{25}}=sqrt{1+3sqrt{1+4sqrt{36}}}=sqrt{1+3sqrt{1+4sqrt{1+5sqrt{49}}}}=sqrt{1+3sqrt{1+4sqrt{1+5sqrt{1+6sqrt{64}}}}}=sqrt{1+3sqrt{1+4sqrt{1+5sqrt{1+6sqrt{1+7sqrt{81}}}}}}$
$=sqrt{1+3sqrt{1+4sqrt{1+5sqrt{1+6sqrt{1+7sqrt{1+8sqrt{1+cdots}}}}}}}$
$5=sqrt{25}=sqrt{1+4sqrt{36}}=sqrt{1+4sqrt{1+5sqrt{49}}}=sqrt{1+4sqrt{1+5sqrt{1+6sqrt{64}}}}=sqrt{1+4sqrt{1+5sqrt{1+6sqrt{1+7sqrt{81}}}}}=sqrt{1+4sqrt{1+5sqrt{1+6sqrt{1+7sqrt{1+8sqrt{100}}}}}}$
$=sqrt{1+4sqrt{1+5sqrt{1+6sqrt{1+7sqrt{1+8sqrt{1+9sqrt{1+cdots}}}}}}}$
$vdots$
$n=sqrt{1+(n-1)sqrt{1+nsqrt{1+(n+1)sqrt{1+(n+2)sqrt{1+(n+3)sqrt{1+(n+4)sqrt{1+cdots}}}}}}}$
$endgroup$
2
$begingroup$
This does not answer the question.
$endgroup$
– Wojowu
23 hours ago
2
$begingroup$
@Wojowu: however, you have to admit that it is a nice piece of information, but too much for a comment. A little explanation might be beneficial though...
$endgroup$
– M.Herzkamp
23 hours ago
add a comment |
$begingroup$
$4=sqrt{16}=sqrt{1+3sqrt{25}}=sqrt{1+3sqrt{1+4sqrt{36}}}=sqrt{1+3sqrt{1+4sqrt{1+5sqrt{49}}}}=sqrt{1+3sqrt{1+4sqrt{1+5sqrt{1+6sqrt{64}}}}}=sqrt{1+3sqrt{1+4sqrt{1+5sqrt{1+6sqrt{1+7sqrt{81}}}}}}$
$=sqrt{1+3sqrt{1+4sqrt{1+5sqrt{1+6sqrt{1+7sqrt{1+8sqrt{1+cdots}}}}}}}$
$5=sqrt{25}=sqrt{1+4sqrt{36}}=sqrt{1+4sqrt{1+5sqrt{49}}}=sqrt{1+4sqrt{1+5sqrt{1+6sqrt{64}}}}=sqrt{1+4sqrt{1+5sqrt{1+6sqrt{1+7sqrt{81}}}}}=sqrt{1+4sqrt{1+5sqrt{1+6sqrt{1+7sqrt{1+8sqrt{100}}}}}}$
$=sqrt{1+4sqrt{1+5sqrt{1+6sqrt{1+7sqrt{1+8sqrt{1+9sqrt{1+cdots}}}}}}}$
$vdots$
$n=sqrt{1+(n-1)sqrt{1+nsqrt{1+(n+1)sqrt{1+(n+2)sqrt{1+(n+3)sqrt{1+(n+4)sqrt{1+cdots}}}}}}}$
$endgroup$
2
$begingroup$
This does not answer the question.
$endgroup$
– Wojowu
23 hours ago
2
$begingroup$
@Wojowu: however, you have to admit that it is a nice piece of information, but too much for a comment. A little explanation might be beneficial though...
$endgroup$
– M.Herzkamp
23 hours ago
add a comment |
$begingroup$
$4=sqrt{16}=sqrt{1+3sqrt{25}}=sqrt{1+3sqrt{1+4sqrt{36}}}=sqrt{1+3sqrt{1+4sqrt{1+5sqrt{49}}}}=sqrt{1+3sqrt{1+4sqrt{1+5sqrt{1+6sqrt{64}}}}}=sqrt{1+3sqrt{1+4sqrt{1+5sqrt{1+6sqrt{1+7sqrt{81}}}}}}$
$=sqrt{1+3sqrt{1+4sqrt{1+5sqrt{1+6sqrt{1+7sqrt{1+8sqrt{1+cdots}}}}}}}$
$5=sqrt{25}=sqrt{1+4sqrt{36}}=sqrt{1+4sqrt{1+5sqrt{49}}}=sqrt{1+4sqrt{1+5sqrt{1+6sqrt{64}}}}=sqrt{1+4sqrt{1+5sqrt{1+6sqrt{1+7sqrt{81}}}}}=sqrt{1+4sqrt{1+5sqrt{1+6sqrt{1+7sqrt{1+8sqrt{100}}}}}}$
$=sqrt{1+4sqrt{1+5sqrt{1+6sqrt{1+7sqrt{1+8sqrt{1+9sqrt{1+cdots}}}}}}}$
$vdots$
$n=sqrt{1+(n-1)sqrt{1+nsqrt{1+(n+1)sqrt{1+(n+2)sqrt{1+(n+3)sqrt{1+(n+4)sqrt{1+cdots}}}}}}}$
$endgroup$
$4=sqrt{16}=sqrt{1+3sqrt{25}}=sqrt{1+3sqrt{1+4sqrt{36}}}=sqrt{1+3sqrt{1+4sqrt{1+5sqrt{49}}}}=sqrt{1+3sqrt{1+4sqrt{1+5sqrt{1+6sqrt{64}}}}}=sqrt{1+3sqrt{1+4sqrt{1+5sqrt{1+6sqrt{1+7sqrt{81}}}}}}$
$=sqrt{1+3sqrt{1+4sqrt{1+5sqrt{1+6sqrt{1+7sqrt{1+8sqrt{1+cdots}}}}}}}$
$5=sqrt{25}=sqrt{1+4sqrt{36}}=sqrt{1+4sqrt{1+5sqrt{49}}}=sqrt{1+4sqrt{1+5sqrt{1+6sqrt{64}}}}=sqrt{1+4sqrt{1+5sqrt{1+6sqrt{1+7sqrt{81}}}}}=sqrt{1+4sqrt{1+5sqrt{1+6sqrt{1+7sqrt{1+8sqrt{100}}}}}}$
$=sqrt{1+4sqrt{1+5sqrt{1+6sqrt{1+7sqrt{1+8sqrt{1+9sqrt{1+cdots}}}}}}}$
$vdots$
$n=sqrt{1+(n-1)sqrt{1+nsqrt{1+(n+1)sqrt{1+(n+2)sqrt{1+(n+3)sqrt{1+(n+4)sqrt{1+cdots}}}}}}}$
edited yesterday
answered yesterday
Okkes DulgerciOkkes Dulgerci
1353
1353
2
$begingroup$
This does not answer the question.
$endgroup$
– Wojowu
23 hours ago
2
$begingroup$
@Wojowu: however, you have to admit that it is a nice piece of information, but too much for a comment. A little explanation might be beneficial though...
$endgroup$
– M.Herzkamp
23 hours ago
add a comment |
2
$begingroup$
This does not answer the question.
$endgroup$
– Wojowu
23 hours ago
2
$begingroup$
@Wojowu: however, you have to admit that it is a nice piece of information, but too much for a comment. A little explanation might be beneficial though...
$endgroup$
– M.Herzkamp
23 hours ago
2
2
$begingroup$
This does not answer the question.
$endgroup$
– Wojowu
23 hours ago
$begingroup$
This does not answer the question.
$endgroup$
– Wojowu
23 hours ago
2
2
$begingroup$
@Wojowu: however, you have to admit that it is a nice piece of information, but too much for a comment. A little explanation might be beneficial though...
$endgroup$
– M.Herzkamp
23 hours ago
$begingroup$
@Wojowu: however, you have to admit that it is a nice piece of information, but too much for a comment. A little explanation might be beneficial though...
$endgroup$
– M.Herzkamp
23 hours ago
add a comment |
Thanks for contributing an answer to Mathematics Stack Exchange!
- Please be sure to answer the question. Provide details and share your research!
But avoid …
- Asking for help, clarification, or responding to other answers.
- Making statements based on opinion; back them up with references or personal experience.
Use MathJax to format equations. MathJax reference.
To learn more, see our tips on writing great answers.
Sign up or log in
StackExchange.ready(function () {
StackExchange.helpers.onClickDraftSave('#login-link');
});
Sign up using Google
Sign up using Facebook
Sign up using Email and Password
Post as a guest
Required, but never shown
StackExchange.ready(
function () {
StackExchange.openid.initPostLogin('.new-post-login', 'https%3a%2f%2fmath.stackexchange.com%2fquestions%2f3119631%2framanujans-radical-and-how-we-define-an-infinite-nested-radical%23new-answer', 'question_page');
}
);
Post as a guest
Required, but never shown
Sign up or log in
StackExchange.ready(function () {
StackExchange.helpers.onClickDraftSave('#login-link');
});
Sign up using Google
Sign up using Facebook
Sign up using Email and Password
Post as a guest
Required, but never shown
Sign up or log in
StackExchange.ready(function () {
StackExchange.helpers.onClickDraftSave('#login-link');
});
Sign up using Google
Sign up using Facebook
Sign up using Email and Password
Post as a guest
Required, but never shown
Sign up or log in
StackExchange.ready(function () {
StackExchange.helpers.onClickDraftSave('#login-link');
});
Sign up using Google
Sign up using Facebook
Sign up using Email and Password
Sign up using Google
Sign up using Facebook
Sign up using Email and Password
Post as a guest
Required, but never shown
Required, but never shown
Required, but never shown
Required, but never shown
Required, but never shown
Required, but never shown
Required, but never shown
Required, but never shown
Required, but never shown
GEWa,YXjFKSBOQPK8vV,ed,5ZXLFbKwnfASp f,hIhcSlMFgAcItkjs,hO8T8aZ pubOc5,j O6Ye7rW1 p1utheyi37YlBYXODWaytQEf
2
$begingroup$
Surely the rigorous definition is that it is the limit of the finite expressions that you get when you drop the $+ cdots$, provided that this limit exists.
$endgroup$
– John Coleman
yesterday
$begingroup$
So you are suggesting $sqrt{1+2sqrt{1+3sqrt{1+4sqrt{1+cdots}}}} $ actually has no universally-agreed definition in Maths?
$endgroup$
– Anson NG
yesterday
6
$begingroup$
When you write something down, you stop your writing at some stage and break into $+ cdots$. What you have written is a purely finite expression. Think of these finite expressions as terms in a sequence. What do these terms approach?
$endgroup$
– John Coleman
yesterday
2
$begingroup$
I understand your comment. Given a general term of partial sum, we can find it limit although it might not exist. We can do it because we all universally agree what it means by a general term. Sometimes the dots is clear in the sense that we would not argue its meaning. For example,comsider $ 1+2+3+4+5+...$. Someone could still argue that the 6th term can be any number and does not have to be 6 but it's kind of agreed that we will look at the pattern and take the 6th term as 6.
$endgroup$
– Anson NG
yesterday
1
$begingroup$
This is interesting since you can redefine any number this way as you pointed out. We need to think of infinite nested root with more careful definition.. Maybe it's only equal to 3 if we don't have to use fraction to expand out more nested root. That is, in the Ramanujan's sum, when you expand the nested root, they are all in term of integers. Where as, for other number, you will get fraction like term. Similar to what you have... just a thought!
$endgroup$
– user209663
yesterday